ADVERTISEMENT
Electrical Shunting Prevents the Decline of Galvanotaxis After Monophasic Pulsed Microcurrent Stimulation in Human Dermal Fibroblasts
Abstract
Background. Electrical stimulation (ES) therapy is recommended for healing pressure injuries. Monophasic pulsed microcurrent stimulation promotes the migration of human dermal fibroblasts (HDFs) to the cathode, and ES potentially accelerates pressure injury healing. A reverse current is generated after ES in the human body; however, the effects of the electrical shunt in preventing the reverse current from migrating are unclear. Therefore, this study aimed to investigate the effects of an electrical shunt on the migration of HDFs.
Methods. In the shunt groups, HDFs were electrically stimulated (0, 200, 400, and 600 µA) for 8 hours, and an electrical shunt was used to remove the electricity after ES. HDFs were observed under time-lapse microscopy for 24 hours. The migration ratio toward the cathode was calculated for each dish.
Results. The migration ratio was significantly higher in the 200-µA group than in the other groups. HDFs migrated toward the anode after ES in the non-shunt groups with greater than 400 µA ES; however, HDFs did not migrate toward the anode with electrical shunting.
Conclusions. A post-ES electrical shunt is important in preventing a decline in the migration effect of ES.
Introduction
Pressure injuries are chronic wounds that deteriorate patients’ quality of life.1 Sanada et al2 reported that the prevalence of pressure injuries in a long-term care hospital was 3.85%, and pressure injuries impose high health care costs in Japan. Pressure injuries of National Pressure Ulcer Advisory Panel Stages 3 and 4 are full-thickness skin loss injuries that require long-term care for wound healing. Therefore, patients with pressure injuries require safe, effective, and low-cost treatments. Electrical stimulation (ES) therapy is recommended for healing pressure injuries, according to the 2019 Pressure Ulcer Prevention & Treatment Quick Reference Guide (recommendation: A).3 Previous reviews have demonstrated the therapeutic effects of ES on pressure injuries.4-7 ES therapy possesses several parameters, such as waveform, voltage, current, frequency, and pulse length. However, the optimum parameters for ES have not been fully elucidated.
The migration, proliferation, and differentiation of human dermal fibroblasts (HDFs) are important factors for granulation-tissue formation, which leads to wound healing.8 Monophasic pulsed microcurrent stimulation (200 µA) promotes migration toward the cathode.9 Furthermore, ES at 200 µA promotes α-SMA expression and contractile ability.10 Based on these results, ES of approximately 200 µA with the cathodic electrodes on the wound site and the anodic electrodes on the adjacent healthy skin accelerates pressure injury healing.11 Thus, 200-µA ES has therapeutic effects on pressure injury. However, several clinical studies have adopted the use of microcurrent greater than 300 µA.12-14 The migration effects of an electrical intensity greater than 300 µA have not been revealed in vitro.
The human body is a known conductor. When the human skin is electrically stimulated, electricity can be stored in the conducting part, and the electric charge may be released after ES as a reverse current with an intensity lower than the flow-current intensity.15 The reverse current potentially affects cell movement. Although HDFs migrate toward the wound site following ES, they may migrate to other sites by reverse currents. Thus, the healing effect of ES may diminish when a reverse current is generated. However, the effect of reverse currents on the migration of HDFs remains unclear. This study hypothesized that HDFs migrate toward the anode after ES, and the migration length toward the cathode decreases without electrical shunting, thus removing the electrical charge after ES. Therefore, our aim was to investigate the effects of electrical shunting on the migration of HDFs using greater than 200-µA monophasic pulsed current stimulation.
Methods and Materials
Reverse-Current Measurement
Ten milliliters of low-glucose Dulbecco’s modified eagle medium (DMEM, Wako Pure Chemicals Industries) in a 100-mm dish was electrically stimulated (intensity: 200 µA, frequency: 2 Hz, and duty factor: 50%) for 10 minutes. In the shunt group, an electrical cable was connected to both electrodes for 1 minute immediately after ES (Figure 1A) to remove the electric charge. Electrical potential differences between the 2 electrodes were measured every minute for 30 minutes after initiating ES to remove the electrical charge.
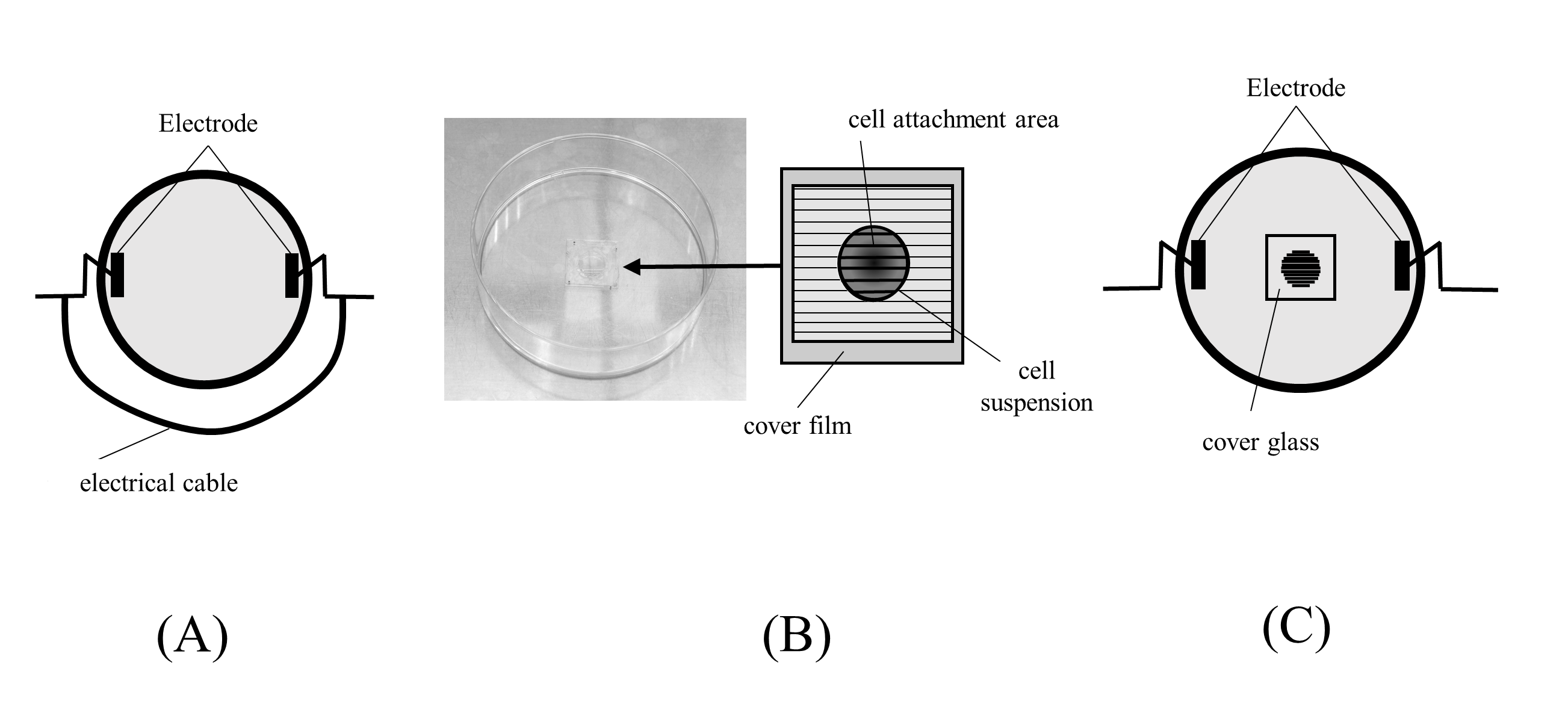
Cells
Primary HDFs (CC-2511; Clonetics) derived from a 33-year-old woman were cultured in a low-glucose DMEM supplemented with 10% fetal bovine serum (Nichirei) in a CO2 incubator at
37 °C. HDFs obtained at passages 7 to 8 were used in this experiment.
Electrical Stimulation
Cell culture was performed as previously described.9 Briefly, cover glasses (CytoGraph, L60S300; Dai Nippon Printing) containing 60 µm-wide grooves for cell attachment were used, and a cover film was placed on each cover glass before cell seeding. HDFs (16.3 × 103 cells) were seeded on each cover glass and cultured in a CO2 incubator at 37 °C (Figure 1B). After 24 hours, the cover film was removed and filled with 10 mL of DMEM. Platinum electrodes were set to conduct electrical currents parallel to the grooves (Figure 1C), and HDFs were electrically stimulated using various currents: 0 µA (control), 200 µA, 400 µA, and 600 µA for 8 hours. A frequency of 2 Hz and duration of 250 ms were used, as in a previous study.9 Electrical shunting was conducted in the shunt group immediately after ES.
Migration Assay
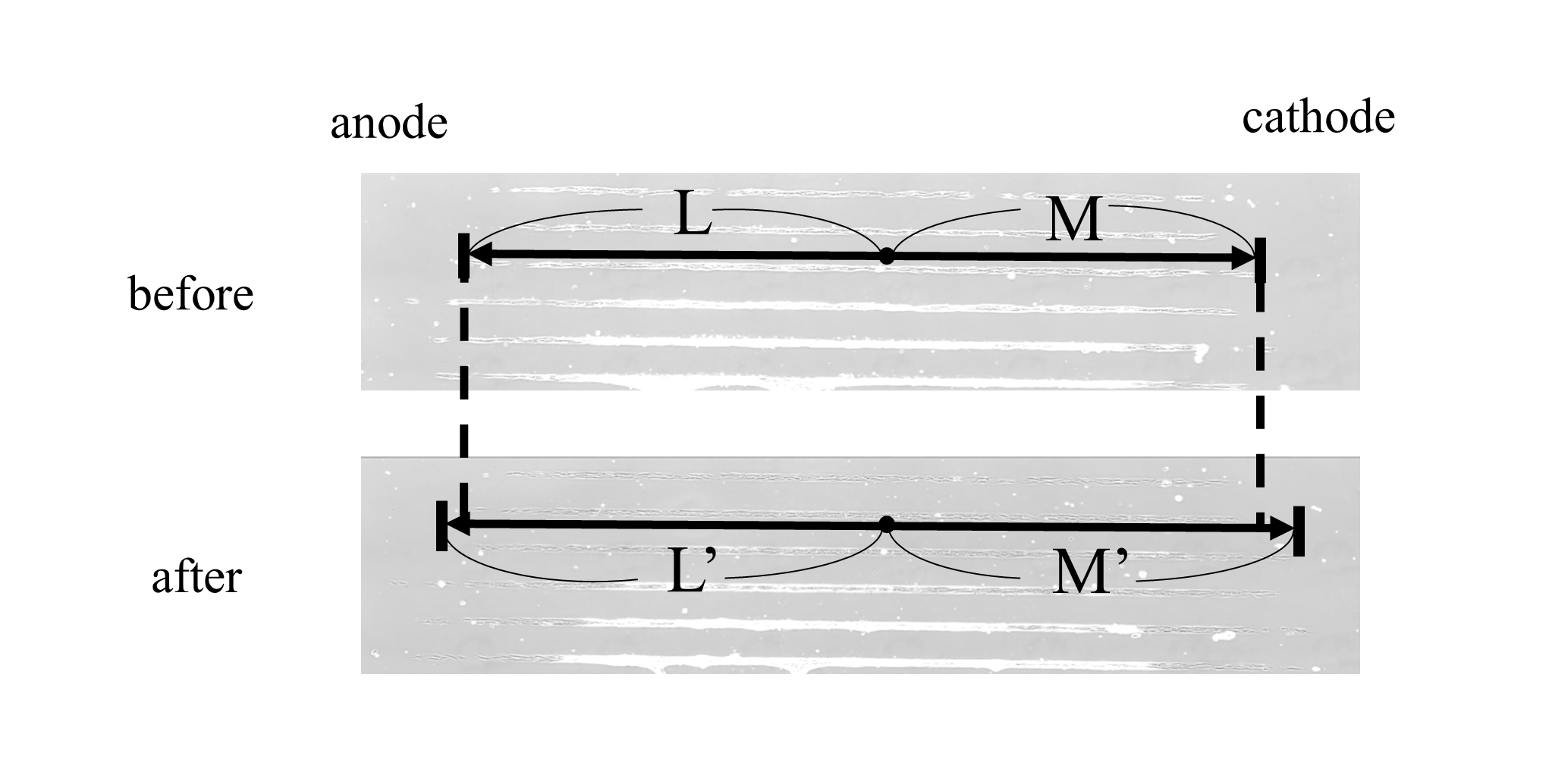
HDFs were observed for 24 hours after initiating ES under time-lapse microscopy (Olympus). The lengths from the standard point marked on each dish to the edge of the cell-adherent area toward the anode (L: before ES and L’: 16 hours after ES) and cathode (M: before ES and M’: after ES) were measured thrice using ImageJ software (US National Institutes of Health). The migration ratio was calculated for each lane as follows: migration ratio = |(M’ - M)/M| / |(L’ - L)/L| (Figure 2). HDFs are considered to migrate toward the cathode more than the anode if the ratio is greater than 1.
Statistical Analysis
Migration-ratio differences between the control and electrically stimulated groups were analyzed using the Tukey–Kramer test after one-way analysis of variance. Migration ratios were analyzed using the paired t test in the shunt and non-shunt groups to assess the shunt’s effect. Results were considered statistically significant at P ≤ .05.
Results
Electrical Potential
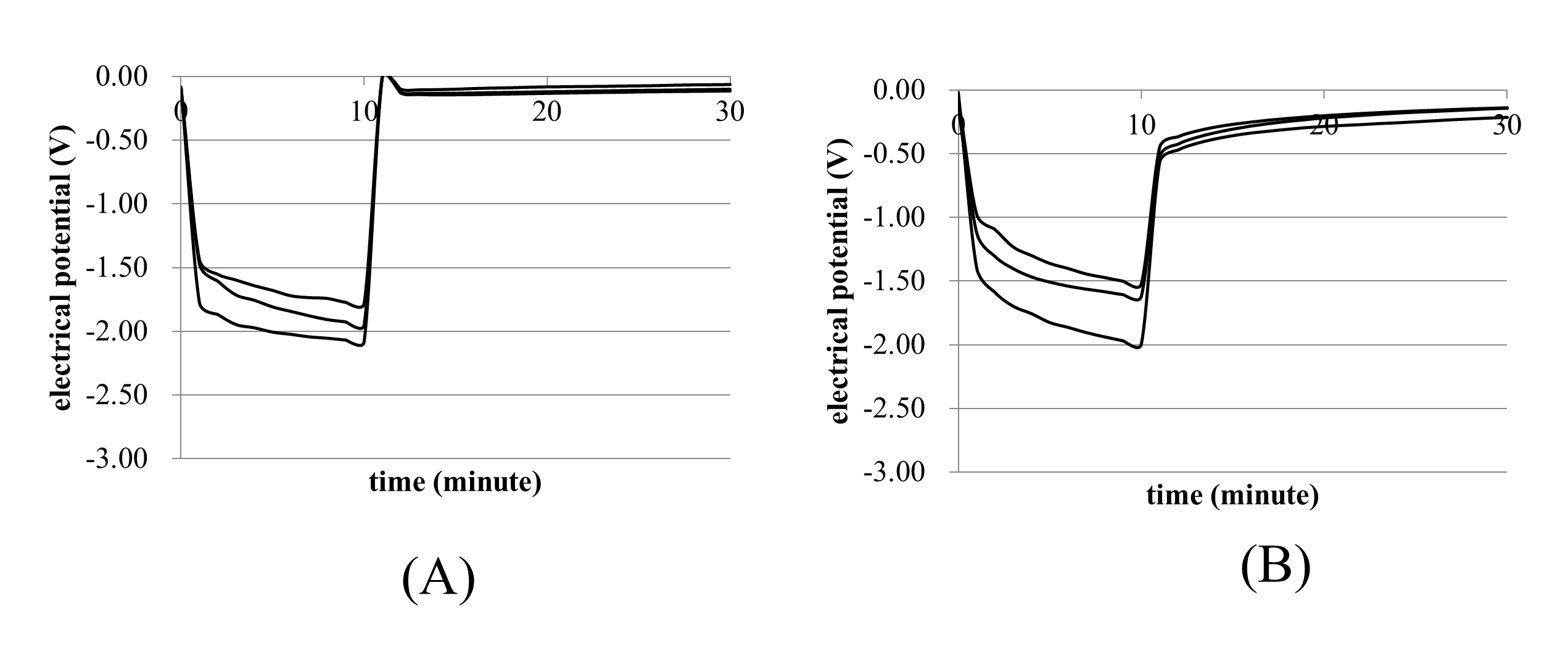
The electrical potentials between the 2 electrodes decreased after initiating ES in the shunt and non-shunt groups (Figure 3A and 3B), and these diminished immediately after ES ceased. Table 1 shows the electrical potentials at 10 (after ES) and 11 minutes (after electrical shunting). Interestingly, there were no significant differences between the shunt and non-shunt groups after ES. However, the electrical potential in the shunt group reached 0 V, whereas that in the non-shunt group did not reach 0 V (0.51 ± 0.05 V). These results suggest that electrical shunting can remove electrical charge and electrical charge discharge after ES without electrical shunting.
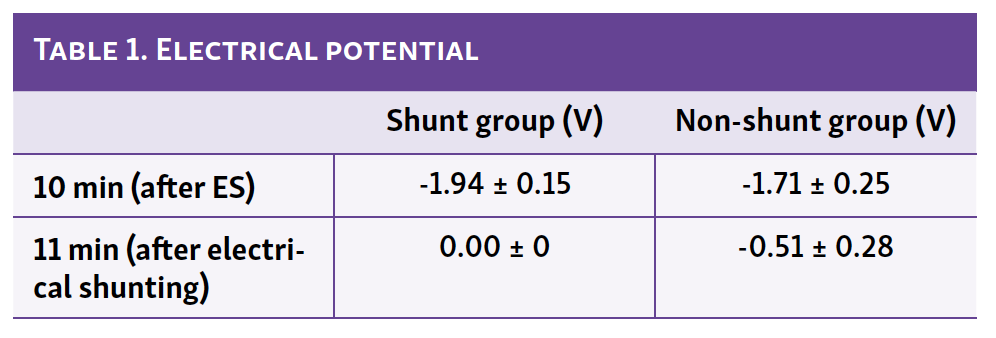
Migration Ratio
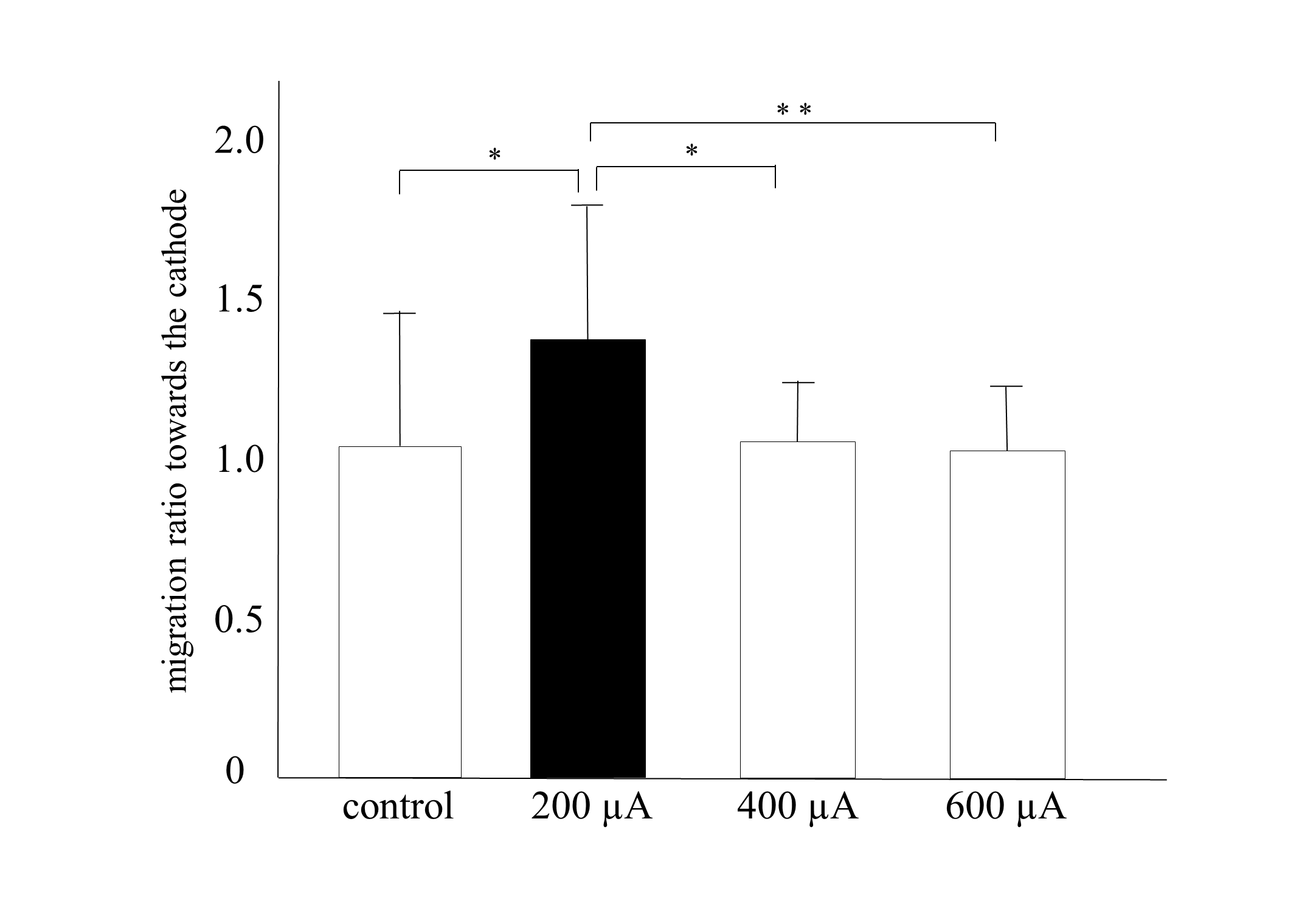
The migration ratio was significantly higher in the 200-µA group than in the other groups (P < .05: vs the control and 400-µA groups; P < .01: vs the 600-µA group; Figure 4). The migration ratios in the 400-µA and 600-µA groups were similar to those in the control group, indicating that an intensity greater than 400 µA did not influence migration. These results suggest that 200 µA is the most effective intensity for migration toward the cathode in HDFs, a finding consistent with those of previous studies.
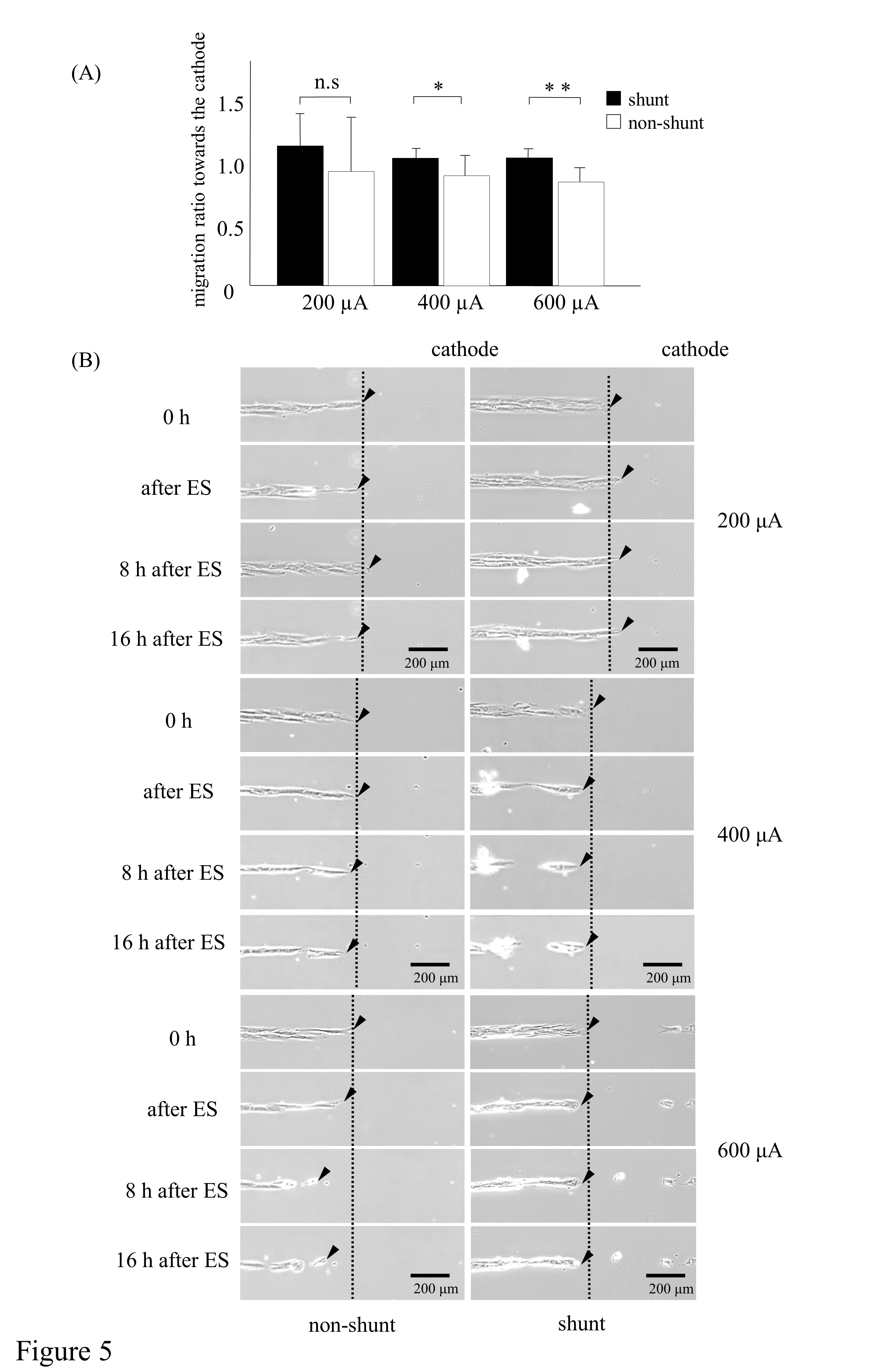
The migration ratios were higher in the shunt groups than in the 400-µA and 600-µA non-shunt groups P < .05: in the 400-µA group; P < .01: in the 600-µA group; Figure 5A, upper). Although the migration ratio was lower in the non-shunt than in the shunt group, there were no significant differences in electrical charge in the 200-µA group. Figure 5B shows that 200-µA electrically stimulated HDFs with electrical shunts migrated toward the cathode with ES, whereas those without an electrical shunt did not. Moreover, the migration of HDFs toward the cathode was not observed in the 400-µA and 600-µA shunt groups (Figure 5B, middle and lower). Furthermore, HDFs migrated in a reverse direction after ES in the non-shunt groups (Figure 5B, middle and lower). These results suggest that the migratory effect of ES is not sufficient without electrical shunting, despite 200-µA ES promoting the migration of HDFs toward the cathode, and an intensity greater than 200 µA has no migratory effect. Contrastingly, HDFs migrated in the opposite direction after ES of similar intensity without electrical shunting.
Discussion
The present study revealed that the reverse current that causes the post-ES migration of HDFs toward the anode can be prevented by electrical shunting. It demonstrated the importance of electrical shunting and removal of electricity to maintain the migration of HDFs following ES.
HDFs did not migrate toward the cathode in the 400-µA and 600-µA shunt groups, despite shunting after ES. Moreover, HDFs migrated toward the anode in the 400-µA and 600-µA non-shunt groups. The electrical potential immediately after ES without shunting was lower than that with shunting, and the potential between the electrodes increased gradually to the initial potential after ES. This suggests that an electric charge was discharged: that is, a reverse current was generated after ES. The intensity of the reverse current is lower than the conducted stimulation in the human body,15 and the current might have promoted the migration of HDFs toward the anode in the 400-µA and 600-µA non-shunt groups. Therefore, the effects of ES on migration potentially decrease without shunting. Accordingly, we speculated that the reverse current affected HDF migration and direction in the non-shunt group.
As previously reported, ES greater than 300 µA promoted pressure injury healing,12-14 and a direct current of 300 µA increased the number of fibroblasts and hydroxyproline levels.16 However, the present study revealed that ES greater than 400 µA does not have a positive effect on the migration of HDFs. Although ES greater than 300 µA potentially promotes the proliferation of HDFs and collagen synthesis in human tissue, leading to wound healing, it is not the optimum intensity for migration. The present study demonstrated that the optimum intensity for promoting migration is 200 µA, and electrical shunting—that is, the removal of electric charge—is necessary to prevent reverse currents and the migration effect of ES. The migration of HDFs from healthy skin toward the wound site is essential for accelerating wound healing because the migration of fibroblasts and myofibroblasts results in wound contraction.17 In this study, the electrical potential dropped immediately after initiating ES. Whereas the electrical potential reached 0 V by electrical shunting, it did not recover sufficiently without electrical shunting. Thus, an electrical charge could be discharged as a reverse current to the initial potential after ES if electrical shunting is not performed. Most studies on electrical stimulation for pressure injury did not consider electrical charge; therefore, reverse current might have affected the migration of HDFs in these studies.
A potential difference is generated when the skin is injured, and an endogenous current—namely, injury current—is generated.18 During normal wound healing, the proliferation phase commences within a few days after the wound occurs, and granulation tissue formation and epithelialization by fibroblasts, endothelial cells, and epithelial cells progress. The potential difference increased 3 days after the wound occurred and decreased as epithelialization progressed.19 The electric potential is lower in the wound area than in normal skin.20 This potential difference is caused by the migration of cells toward the wound area. Moreover, cell migration and wound healing are promoted by the generation of potential differences with exogenous ES.21 However, the reverse current may reduce the therapeutic effect of ES because the current flows from the wound toward the normal skin surrounding the wound. In this study, the migration ratio of HDFs decreased without electrical shunting.
Limitations
The electrical potential following ES in vitro was measured; however, the actual intensity of the reverse current was not clear. Furthermore, future studies are required to investigate the difference in electric potential following ES with/without electrical shunting in chronic wounds. The present study revealed the importance of electrical shunting and the optimum intensity for migration. It is important to reveal the effects of injury current, skin electrical impedance, and intensity control on wound healing to apply to the clinical trials.
Conclusions
This study examined the effect of electrical shunting on HDFs migration after ES. It found that an intensity greater than 400 µA is not suitable for promoting the migration of HDFs, and electrical shunting potentially prevents the reverse migration of HDFs after ES. The present study suggests that it is important to remove electricity after ES to prevent the generation of reverse currents when ES is applied to promote migration of HDFs.
Acknowledgments
The authors would like to thank Editage [http://www.editage.com] for editing and reviewing this manuscript for English language.
Affiliations: 1Kansai University of Welfare Sciences, Faculty of Health Science, Department of Rehabilitation, Osaka, Japan; 2Toyama Rehabilitation Medical Health and Welfare College, Toyama, Toyama; 3Naragakuen University, Faculty of Heath Science, Department of Rehabilitation, Ikoma, Nara; 4Department of Rehabilitation, Yoshida Hospital, Kobe, Japan
Correspondence: Mikiko Uemura, PhD, PT; mic.uem@gmail.com
Funding: This study was partially supported by the Japan Society for the Promotion of Science (JSPS) KAKENHI (Grant Number: 16K01529).
Ethics: This study used a cell culture supplied by Clonetics and therefore did not require ethical approval.
Disclosures: The authors have no conflicts of interest to declare.
References
1. Ohura T, Sanada H, Mino Y. Clinical activity-based cost effectiveness of traditional versus modern wound management in patients with pressure ulcers. Wounds. 2004;16(5):157-163.
2. Sanada H, Miyachi Y, Ohura T, et al. The Japanese pressure ulcer surveillance study: a retrospective cohort study to determine prevalence of pressure ulcers in Japanese hospitals. Wounds. 2008;20(6):176-182.
3. National Pressure Ulcer Advisory Panel (NPUAP), European Pressure Ulcer Advisory Panel (EPUAP), and Pan Pacific Pressure Injury Alliance (PPPIA). Prevention and Treatment of Pressure Ulcers: Clinical Practice Guideline. National Pressure Ulcer Advisory Panel. 2019. Accessed December 5, 2021. https://www.internationalguideline.com/guideline.
4. Hunckler J, de Mel A. A current affair: electrotherapy in wound healing. J Multidiscip Healthc. 2017;10:179-194. Published 2017 Apr 20. doi:10.2147/JMDH.S127207
5. Kawasaki L, Mushahwar VK, Ho C, Dukelow SP, Chan LL, Chan KM. The mechanisms and evidence of efficacy of electrical stimulation for healing of pressure ulcer: a systematic review. Wound Repair Regen. 2014;22(2):161-173. doi:10.1111/wrr.12134
6. Lala D, Spaulding SJ, Burke SM, Houghton PE. Electrical stimulation therapy for the treatment of pressure ulcers in individuals with spinal cord injury: a systematic review and meta-analysis. Int Wound J. 2016;13(6):1214-1226. doi:10.1111/iwj.12446
7. Kloth LC. Electrical stimulation technologies for wound healing. Adv Wound Care (New Rochelle). 2014;3(2):81-90. doi:10.1089/wound.2013.0459
8. Schreier T, Degen E, Baschong W. Fibroblast migration and proliferation during in vitro wound healing. A quantitative comparison between various growth factors and a low molecular weight blood dialysate used in the clinic to normalize impaired wound healing. Res Exp Med (Berl). 1993;193(4):193-205. doi:10.1007/BF02576227
9. Uemura M, Maeshige N, Koga Y, et al. Monophasic pulsed 200-µA current promotes galvanotaxis with polarization of actin filament and integrin α2ß1 in human dermal fibroblasts. Eplasty. 2016;16:e6. Published 2016 Jan 19.
10. Uemura M, Sugimoto M, Yoshikawa Y, Hiramatsu T, Inoue T. Monophasic pulsed current stimulation of duty cycle 10% promotes differentiation of human dermal fibroblasts into myofibroblasts. Phys Ther Res. 2021;24(2):145-152. Published 2021 Mar 18. doi:10.1298/ptr.E10064
11. Hiramatsu T, Sugimoto M, Yoshikawa Y, Uemura M, Mori Y, Icihhori R. Randomized double-blind cross over trials for the effects of electrical stimulation therapy for pressure injuries healings. Wound Rep Reg. 2020;28(5):A12.
12. Wood JM, Evans PE 3rd, Schallreuter KU, et al. A multicenter study on the use of pulsed low-intensity direct current for healing chronic stage II and stage III decubitus ulcers. Arch Dermatol. 1993;129(8):999-1009.
13. Carley PJ, Wainapel SF. Electrotherapy for acceleration of wound healing: low intensity direct current. Arch Phys Med Rehabil. 1985;66(7):443-446.
14. Cukjati D, Robnik-Sikonja M, Rebersek S, Kononenko I, Miklavcic D. Prognostic factors in the prediction of chronic wound healing by electrical stimulation. Med Biol Eng Comput. 2001;39(5):542-550. doi:10.1007/BF02345144
15. Rothwell JC, Hallett M, Berardelli A, Eisen A, Rossini P, Paulus W. Magnetic stimulation: motor evoked potentials. The International Federation of Clinical Neurophysiology. Electroencephalogr Clin Neurophysiol Suppl. 1999;52:97-103.
16. Demir H, Balay H, Kirnap M. A comparative study of the effects of electrical stimulation and laser treatment on experimental wound healing in rats. J Rehabil Res Dev. 2004;41(2):147-154. doi:10.1682/jrrd.2004.02.0147
17. Gabbiani G. The cellular derivation and the life span of the myofibroblast. Path Res Pract. 1996;192(7):708-711. doi:10.1016/S0344-0338(96)80092-6
18. Baker AT, Jaffe LF, Vanable JW Jr. The glabrous epidermis of cavies contains a powerful battery. Am J Phyisol. 1982;242(3):R358-R366. doi:10.1152/ajpregu.1982.242.3.R358
19. Dubé J, Rouchette-Drouin O, Lévesque P, et al. Restoration of the transepithelial potential within tissue-engineered human skin in vitro and during the wound healing process in vivo. Tissue Eng Part A. 2010;16(10):3055-3063. doi:10.1089/ten.TEA.2010.0030
20. Moulin VJ, Dubé J, Rochette-Drouin O, et al. Electric potential across epidermis and its role during wound healing can be studied by using an in vitro reconstructed human skin. Adv Wound Care (New Rochelle). 2012;1(2):81-87. doi:10.1089/wound.2011.0318
21. Luo R, Dai J, Zhang J, Li Z. Accelerated skin wound healing by electrical stimulation. Adv Healthc Mater. 2021;10(16):e2100557. doi:10.1002/adhm.202100557