Classification of Burn Depth
Questions
1. What is the theoretical and practical significance of classifying the depth of a burn?
2. What are the zones of injury?
3. What is happening biochemically in the zone of stasis?
4. How can the zone of stasis be restrained?
Case Description
A 41-year-old man was seen in the emergency department after his shirt caught fire while cooking. He sustained a 15% total body surface area burn localized to his right upper extremity and chest. There was no evidence of inhalation injury. Fragile blisters were seen on the forearm. These were debrided along with loose devitalized tissue to reveal an area of white and insensate skin. This deep area was excised and skin grafted. The adjacent skin was red and blanched when touched. This superficial aspect of the burn healed spontaneously within 14 days.
Video. Clinical assessment of mixed thickness burns.
Q1. What is the theoretical and practical significance of classifying the depth of a burn?
Historically, burns have been classified by the extent of penetration into the tissues as degrees (Figures 1 and 2). The number of divisions has varied over the centuries and has included the 6-degree classification of Dupuytren and the more popular and current 3-degree systems that resemble that described by Wilhelm Fabricius Hildanus in his monograph of 1610. He defined “three degrees” as: (1) “…reddened skin, very sharp piercing pain as if stung by a nettle… (2) …the affected area becomes swollen and blisters are raised … (3) …the skin is black … and if pierced with a scalpel there is a hard dry crust that peels off to leave a deep festering sore.”1
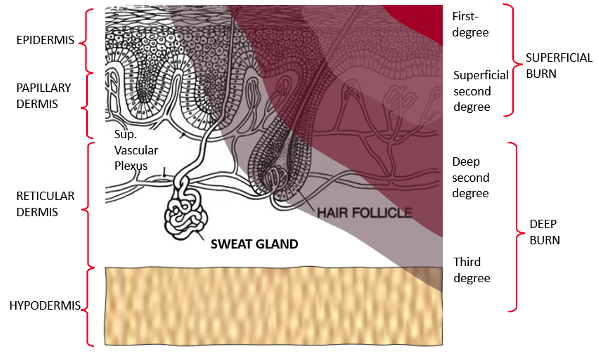
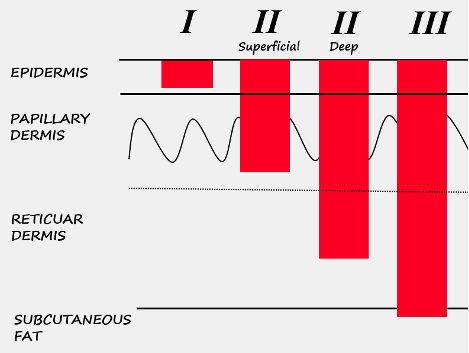
The current first-, second-, and third-degree classification corresponds to extension of the burn into the epidermis, dermis, or subcutaneous fat, respectively. Burns extending into deeper structures, such as muscle or bone, are typically classified as fourth-degree. Although there is no clear line of demarcation, the dermis is divided into the papillary dermis and the reticular dermis (Figure 1).
Since the dermis contains the highest concentration of reparative cells, the capacity for burns to spontaneously re-epithelialize depends largely on the volume of dermis spared. Derganc in 1953 led the way in subdividing second-degree burns into superficial and deep categories (which he called II.A and II.B) that better relate to expected functional outcomes and treatment2 (Figure 2).3
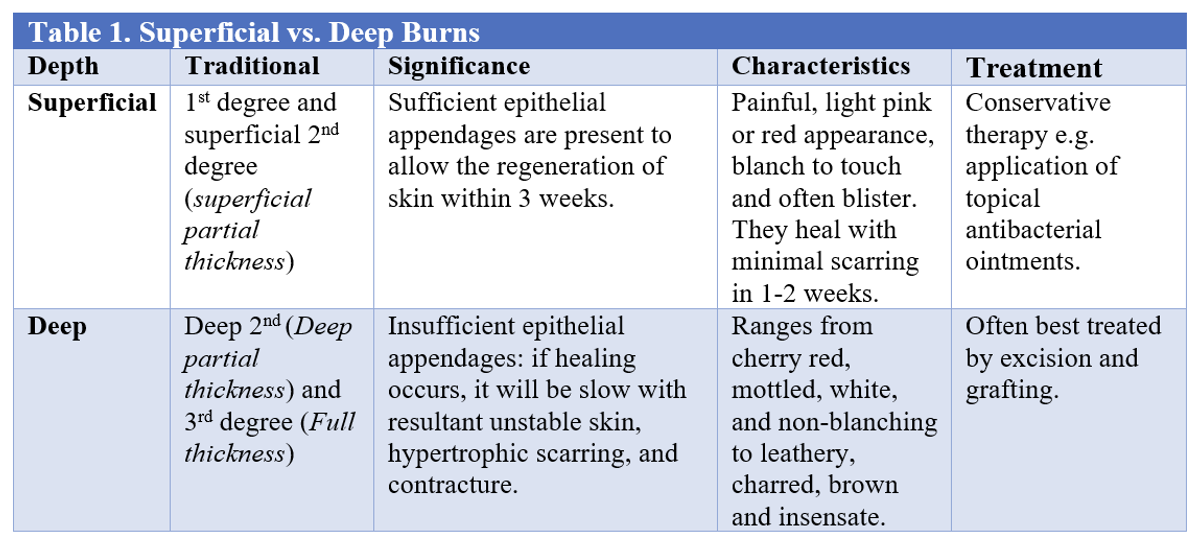
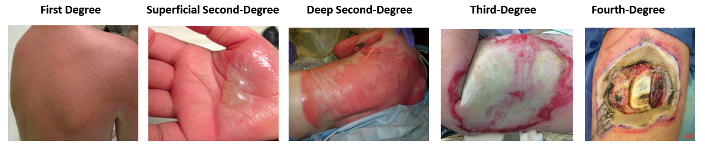
Superficial burns, which include first-degree and superficial second-degree injuries, result in the rapid regeneration of the epidermis from the intact basal layer or from the abundant epithelial appendages in the papillary dermis within 1-3 weeks. Conversely, deep burns, which comprise deep second-degree and third-degree wounds, have insufficient epithelial appendages and therefore longer healing times. The dermis contains 2 separate fibroblast lineages, which respond differently to microenvironmental niche signals. The papillary fibroblasts mediate non-fibrotic wound repair, whereas reticular fibroblasts cause fibrosis and scarring.4 Third-degree burns may only heal if small enough to allow re-epithelialization from the wound edges. The clinical features and treatment are depicted in Table 1 and Figure 3.
Q2. What are the zones of injury?
Jackson in 1953 conceptualized the burn wound as a 3-dimensional mass of tissue with 3 concentric zones of injury, “hyperemia, stasis of capillary flow, and coagulation”5 (Figure 4). However, it is important to realize that burns are dynamic.
The zone of coagulation is an irreversible area of necrosis nearest the heat source. Conversely, the peripheral zone of hyperemia has increased perfusion due to vasodilatory inflammatory edema, seen microscopically as loss of epidermis with intact subpapillary plexus and capillary loops. The skin is therefore red, blanches on pressure, may blister, and usually re-epithelializes within a week (Video). Sandwiched between these 2 regions lies the zone of stasis, which experiences poor perfusion and is initially red but may blanche with pressure because the subpapillary plexus is intact. Cellular damage in this region may be salvageable, although there is a tendency for the wound to deepen within the next 24 to 48 hours, a process termed “burn wound conversion.” The superficial capillaries become clogged with red cells and microthrombi that exacerbate the inflammatory response. The skin becomes mottled red and white, clinically indistinguishable from the zone of coagulation (Figure 5). The consequent increase in the size and depth of the burn increases the need for skin grafting and the risk of sepsis, shock, hypertrophic scarring, and death. Therefore, the objectives of treating burn wounds are to rescue the tissue in the zone of hyperemia, accelerate the recovery of tissue in the zone of stasis, and remove eschar in the zone of necrosis.
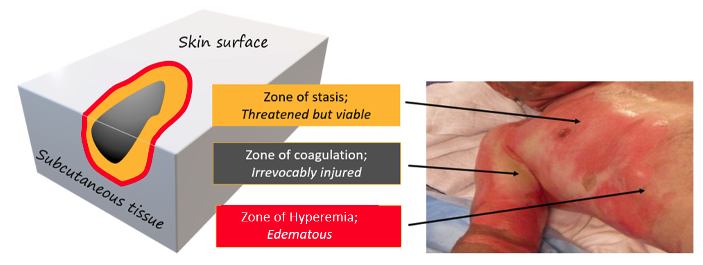
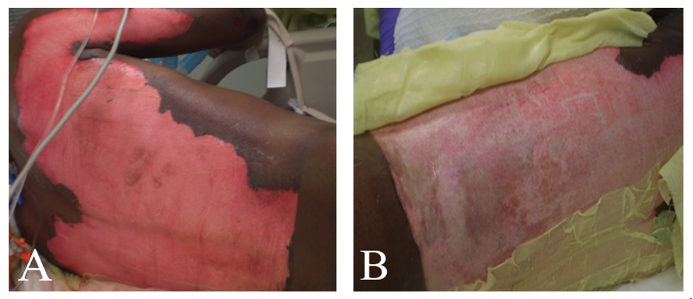
Q3. What is happening biochemically in the zone of stasis?
The processes involved in the progression of partial-thickness burn wounds to full-thickness necrosis include inflammation, lipid peroxidation of cell membranes, and depletion of adenosine triphosphate (ATP). Severe burns activate nuclear factor κB (NF-κB), a family of inducible transcription factors that regulate the induction of proinflammatory cytokines, including tumor necrosis factor-α (TNF-α) and interleukin-6 (IL-6).6 These cytokines induce the production of inflammatory mediators by macrophages. The imbalance between vasoconstrictive and vasodilatory prostanoids impairs tissue perfusion, which is exacerbated by histamine, bradykinin, and products of complement activation that promote capillary permeability and create edema-related fluid shifts (Figure 6).7 Tissue ischemia may be further augmented by delayed escharotomy, hypovolemia, and desiccation of the wound.8
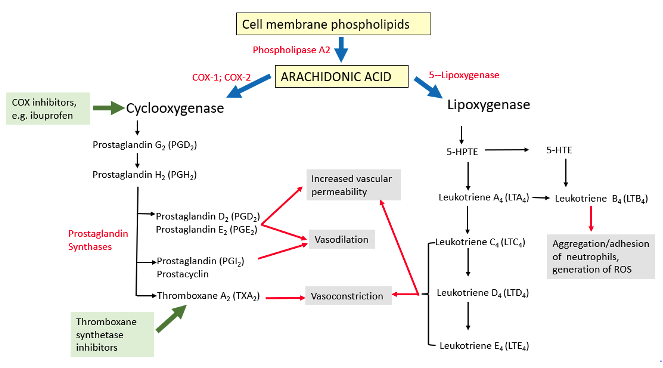
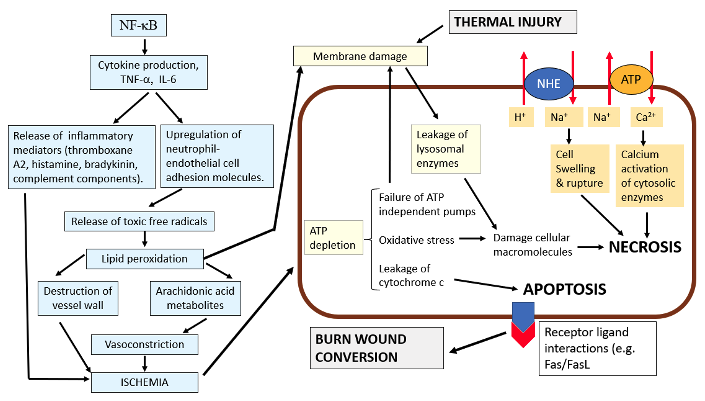
Cytokines also promote the interaction of integrins (eg, CD11/CD18) on neutrophils with adhesion molecules (eg, ICAM-1/VCAM-1) on the endothelial cells of post-capillary venules, generating reactive oxygen and nitrogen species including the highly reactive hydroxyl (OH*) radical. The resulting lipid peroxidation destroys cell membranes, a process compounded by the disparity between oxidants and antioxidants. Lysosomal damage and leakage of hydrolytic enzymes exacerbate the necrosis (Figure 7).
ATP depletion arises from ischemic conditions, leading to reduced oxidative phosphorylation within the mitochondrial electron transport system. Concurrently, DNA strand breaks activate poly (ADP-ribose) polymerase (PARP-1), depleting its substrate NAD+ upon which the generation of ATP depends. Despite anaerobic metabolism, insufficient ATP is synthesized to fuel the ATPase-dependent transport systems (eg, Na+/K+ ATPase, Ca2+ ATPase). This insufficiency results in lactic acidosis, triggering the extrusion of H+ ions in exchange for Na+ ions. The elevated intracellular Na+ levels disrupt cellular volume regulation, causing osmotic swelling and eventual rupture.9 An influx of Ca2+ ions in exchange for Na+ ions activate cytosolic enzymes phospholipases, proteases, and endonucleases that fragment membranes, cytoskeletal proteins, and DNA, respectively. Leakage of cytochrome C from the mitochondria activates caspases and promotes apoptosis, a process also induced by the interaction of plasma membrane death receptors such as FAS on basal keratinocytes and its ligand FasL.10
Q4. How can the zone of stasis be restrained?
Current clinical strategies for preventing conversion of the burn wound include ensuring adequate fluid resuscitation to restore perfusion and the removal of surface eschar, bacteria, and neutrophils to decrease the level of oxidants and proteases in the wound. Additionally, biological dressings such as xenograft and allograft that counter desiccation have been shown to improve wound healing. Various other methods have been explored, including the use of TNF-α inhibitors, antioxidants (eg, catalase, superoxide dismutase, and vitamin C), heparin, steroidal and nonsteroidal medications, thromboxane inhibitors,7 epidermal growth factor, monoclonal antibodies against membrane glycoprotein CD-18 (to block neutrophil adhesion to microvascular endothelium), hypothermia, hyperbaric oxygen, erythropoietin (an anti-inflammatory, anti-apoptotic cytokine that promotes vasodilation and angiogenesis), and negative pressure wound therapy.11 These have demonstrated the ability to augment dermal perfusion in experimental settings, but their clinical role remains unconfirmed.
Acknowledgments
Affiliation: Professor of Plastic and Reconstructive Surgery, Johns Hopkins University School of Medicine, Baltimore, MD (Ret.)
Correspondence: Stephen M. Milner, MBBS, BDS, DSc (Hon), FRCSE, FACS; stephenmilner123@gmail.com
Disclosures: The author discloses no relevant conflict of interest or financial disclosures for this manuscript.
References
1. Wallace A. Classification of Burns: A History of Development; with Comments for Today and Thoughts for the Future. Thesis PhD. University of St Andrews; 1973.
2. Derganc M. The theoretical and practical significance of the subdivision of the second degree burn into superficial and deep type. In: Artz CP, ed. Research in Burns. Vol 9. American Institute of Biological Sciences and F A Davis; 1962:315-326.
3. Klasen HJ. Classification of burns. In: History of Burns. Erasmus Publishing; 2004:33-66.
4. Mascharak S, desJardins-Park HE, Longaker MT. Fibroblast heterogeneity in wound healing: Hurdles to clinical translation. Trends Mol Med. 2020;26(12):1101-1106. doi:10.1016/j.molmed.2020.07.008
5. Jackson DM. The diagnosis of the depth of burning. Br J Surg. 1953;40(164):588-596. doi:10.1002/bjs.18004016413
6. Liu T, Zhang L, Joo D, Sun SC. NF-κB signaling in inflammation. Signal Transduct Target Ther. 2017;2:17023. doi:10.1038/sigtrans.2017.23
7. DelBeccaro EJ, Robson MC, Heggers JP, Swaminathan R. The use of specific thromboxane inhibitors to preserve the dermal microcirculation after burning. Surgery. 1980;87(2):137-141.
8. Singh V, Devgan L, Bhat S, Milner SM. The pathogenesis of burn wound conversion. Ann Plast Surg. 2007;59(1):109-115. doi:10.1097/01.sap.0000252065.90759.e6
9. Kalogeris T, Baines CP, Krenz M, Korthuis RJ. Ischemia/reperfusion. Compr Physiol. 2016;7(1):113-170. doi:10.1002/cphy.c160006
10. Shupp JW, Nasabzadeh TJ, Rosenthal DS, Jordan MH, Fidler P, Jeng JC. A review of the local pathophysiologic bases of burn wound progression. J Burn Care Res. 2010;31(6):849-873. doi:10.1097/BCR.0b013e3181f93571
11. Palackic A, Jay JW, Duggan RP, et al. Therapeutic strategies to reduce burn wound conversion. Medicina (Kaunas). 2022;58(7):922. doi:10.3390/medicina58070922