Alternative Antimicrobial Delivery Options for Diabetic Foot Infections
The management of diabetic foot infections is a significant challenge in healthcare, often complicated by their polymicrobial nature and the rising incidence of antibiotic resistance.1 Traditional oral and intravenous antibiotics occasionally fail to deliver effective results due to poor penetration at the infection site, necessitating alternative strategies.
One such promising approach to consider is the use of drug-eluting materials (DEMs). These materials provide localized and sustained release of therapeutic agents directly at the wound site, supplementing systemic antibiotic therapy and potentially reducing the overall antibiotic burden.2 This article explores the development, properties, and applications of DEMs and their impact on healthcare costs.
The Treatment Approach to Diabetic Foot Infections
Diabetic foot wounds often harbor complex communities of microorganisms, including bacteria, fungi, and viruses, which can form biofilms that protect them against the host immune response and antibiotics. Biofilms are especially problematic as they can increase bacterial resistance to antibiotics by up to 1000 times, making infections exceedingly difficult to treat.3 The presence of biofilms can also impede wound healing by creating a persistent inflammatory environment and providing a reservoir for reinfection.4
Given the polymicrobial nature of these infections, a multifaceted treatment approach is often necessary. This approach combines systemic therapies, such as oral or intravenous antibiotics, with localized treatments, such as debridement and the application of antimicrobial dressings or drug-eluting materials.5 Such comprehensive strategies are essential to effectively eradicate the pathogens, break down biofilms, and promote wound healing, ultimately improving patient outcomes and reducing the risk of complications.6
Drug-Eluting Materials as a Supplement to Oral and Intravenous Antibiotics
Drug-eluting materials (DEMs) offer a promising supplement to traditional oral and intravenous antibiotic treatments. These materials are designed to release therapeutic agents directly at the site of infection in a controlled manner over an extended period. This localized delivery allows for higher drug concentrations at the wound site compared to systemic administration, enhancing treatment efficacy and reducing systemic side effects. Furthermore, by targeting the site directly, DEMs can help overcome the challenges posed by biofilms and antibiotic resistance, both significant barriers to effective treatment.7
The concept of DEMs dates back to the mid-20th century with early applications in cardiovascular medicine. Developing drug-eluting stents, for instance, helped prevent restenosis after angioplasty by releasing antiproliferative drugs. Adaptations later emerged in wound care, orthopedics, and other medical fields. Over the years, scientists have made significant advancements in the materials used, the drugs incorporated, and the delivery mechanism, leading to more effective and versatile drug-eluting products.5
Ideal Properties of Eluting Materials
In order for DEMs to be effective in wound management, they must possess several key properties:8
Biocompatibility. The material must not elicit an adverse immune response.
Controlled drug release. The material should release the drug at a consistent and predictable rate to maintain therapeutic levels over the required duration.
Mechanical stability. The material must be durable and maintain its integrity throughout the treatment period.
Ease of application. The material must be easy to apply and conform to the wound.
Antimicrobial efficacy. The drug released should be effective against a broad spectrum of pathogens including those in biofilms.
Osteoconductive. The material must encourage new bone overgrowth and remodeling.
Current Drug-Eluting Products and Uses
A widely used DEMs is antibiotic-loaded bone cement, particularly polymethyl methacrylate (PMMA), which also has use in joint arthroplasty, trauma and spine surgery. PMMA is particularly valuable in treating osteomyelitis that can be difficult to eradicate with systemic antibiotics alone. The local delivery of antibiotics through PMMA cement can allow for high drug concentrations at the infection site, enhancing therapeutic outcome. Additionally, this approach may help to prevent the development of antibiotic resistance by reducing the need for prolonged systemic antibiotics.
In a retrospective study, Dai and colleagues found that compared with a control group, the group treated with antibiotic-loaded PMMA bone cement had fewer debridements and a shorter duration of wound healing (35.32 ± 3.77 days vs. 44.37 ± 7.44 days, P < .001).9
Applying antibiotic-loaded bone cement proved to be an effective solution for treating infected diabetic foot ulcers (DFUs), significantly decreasing the frequency of debridement procedures and shortening the healing duration in patients with infected DFUs. This evidence supports the use of PMMA as a valuable tool in managing complex wound infections, offering a practical and effective means of enhancing patient outcomes and combating the persistent issue of antibiotic resistance.
Morley and colleagues conducted a retrospective review of 137 patients with either osteomyelitis or soft tissue infection, all of whom underwent surgical debridement with placement of local antibiotic-loaded calcium sulfate. As a result, 88.3% of infections resolved and 82.5% of wounds healed on an average of 11.3 weeks.10 Further, 22 patients experienced infection eradication without using postop systemic antibiotics. Similarly, Gauland found that in 323 patients with lower extremity osteomyelitis treated with surgical debridement and implanted antibiotic calcium sulfate tablets, 279 patients healed clinically without IV antibiotics, 24 patients healed with IV antibiotics, and 20 required amputation.11
In another retrospective review, Elmarsafi and colleagues assessed outcomes of permanent antibiotic-eluting cement spacers in 30 patients.12 As a result, spacers were either retained or exchanged in 20 patients. The remaining 10 experienced spacer failure that required removal. Additionally, the longest duration for a retained spacer was 76 months, while the longest duration for an exchanged spacer was 111 months at the last follow-up.
Drug delivery systems have seen significant improvements in bone cements, enhancing degradation rates and effectiveness as local drug carriers for antibiotics, antimicrobial peptides, and other therapies. New formulations incorporate core materials such as calcium sulfate, calcium phosphate, PMMA, and bioactive glasses.13 In a study by Boyle and colleagues, an implantable calcium phosphate–calcium sulfate mixture exhibited similar antibacterial efficacy to PMMA.14 Additionally, this mixture demonstrated partial resorption, unlike PMMA, which is non-resorbable and has a higher likelihood of bacterial colonization and less mechanical strength.
Case Studies in Antimicrobial Delivery for DFUs
Case 1. A 61-year-old female with a past medical history of diabetes with neuropathy and chronic kidney disease presented with chronic ulcer of the right foot. The wound probed deep to bone and radiographs demonstrated concern for osteomyelitis of the fourth metatarsal head. She subsequently underwent bone debridement of the fourth metatarsal with wound closure and placement of antibiotic-impregnated beads of the right foot. Pathology results did not reveal any definitive acute osteomyelitis to the fourth metatarsal bone. At 6 weeks postoperatively, the surgical site was completely healed (Figure 1D).
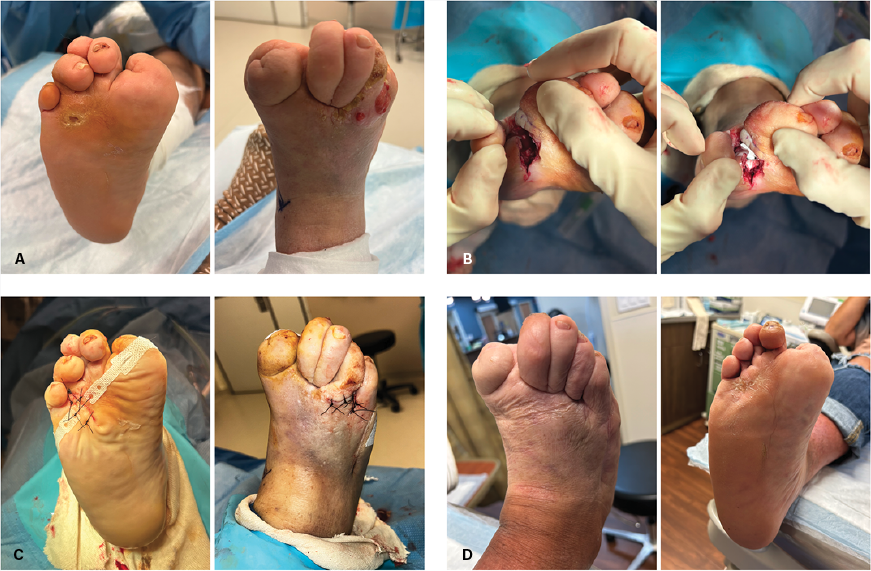
Case 2. A 73-year-old male with type 2 diabetes and polyneuropathy presented with a wound to the right fifth metatarsal base with exposed bone (Figure 2A). Radiographs demonstrated bony destruction to the area and magnetic resonance images (MRI) demonstrated loss of normal T1 marrow signal with enhancement involving a majority of the fifth metatarsal, sparing the metatarsal head.
Given these concerns for infection, he underwent excisional debridement to the base of the right fifth metatarsal as well as cuboid biopsy. Surgeons packed the surgical site open. The patient underwent repeat bone debridement 3 days later with placement of calcium sulfate antibiotic-impregnated beads and delayed closure (Figure 2B). Bone pathology at the fifth metatarsal base and cuboid revealed acute osteomyelitis. The patient subsequently had a 6 weeks of antibiotics.
At 2 months postop, the incision appeared clean and dry with skin edges mostly coapted (Figure 2C). Physicians removed sutures during this visit and reinforced the surgical site with adhesive strips to maintain skin approximation. At 4 months postop, the surgical site was completely epithelialized with no open lesions (Figure 2D).
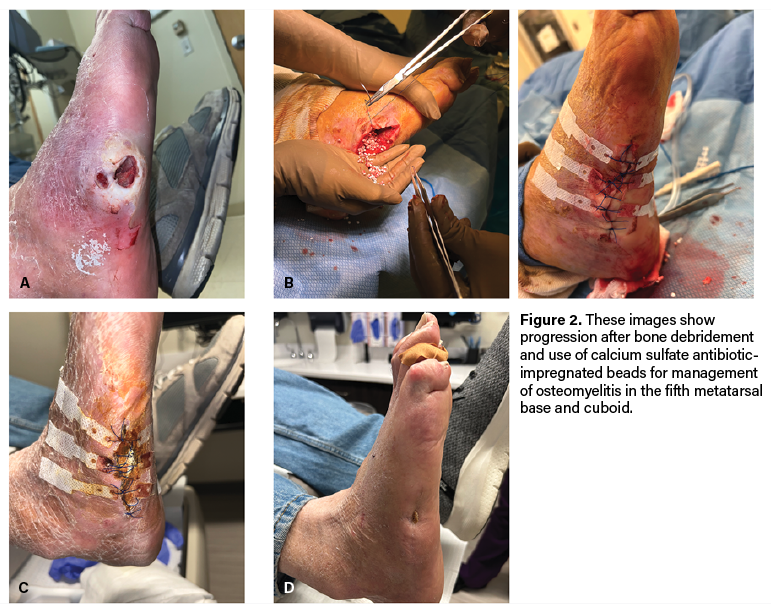
How DEMs Can Positively Impact Costs and Outcomes
While DEMs initially incur higher costs compared to standard treatments, their long-term benefits could yield significant cost savings by reducing infection rates, complications, and the need for additional surgeries and prolonged hospital stays. For instance, antibiotic-loaded PMMA bone cement has demonstrated effectiveness in lowering the incidence of deep infections following orthopedic procedures, thus alleviating the economic burden associated with treating these complications.9
The use of DEMs may also contribute to improved patient outcomes, such as faster wound healing and reduced morbidity. This not only enhances patient quality of life by potentially shortening hospital stays and minimizing discomfort, but also may contribute to optimizing healthcare efficiency by reducing intervention frequency and medical consultations. By preventing infections and their associated complications, DEMs help in conserving healthcare resources and redirecting them towards other critical areas of patient care.
Research indicates that applying antibiotic-loaded bone cement in diabetic foot ulcer management leads to significant reductions in treatment costs by minimizing the need for multiple surgeries and extended antibiotic courses.15 This cost-effectiveness extends beyond direct medical expenses to encompass indirect costs associated with prolonged hospitalizations and rehabilitation, thereby offering a comprehensive approach to managing chronic wounds and complex infections.
The Future of Drug-Eluting Biocomposites
The future of DEMs is promising with ongoing research and development aimed at enhancing their efficacy and expanding their applications. Potential future directions include the development of DEMs that can respond to environmental triggers such as changes in pH or temperature. Additionally, the integration of multiple therapeutic agents—such as antibiotics, growth factors, and anti-inflammatory drugs—into one single material could also offer a more comprehensive approach to wound management.
Bacteriophages also present an innovative approach for treating long-term, antibiotic-resistant infections through bacterial lysis. Phages proliferate in the presence of bacterial hosts, allowing for more controlled dosage amounts.13 Past studies have demonstrated that bacteriophage SAP-26 has bactericidal activity against over 1000 methicillin-resistant Staphylococcus aureus (MRSA) strains and 56 methicillin-susceptible Staphylococcus aureus (MSSA) strains. When combined with antibiotics, bacteriophages reduced bacterial biofilm by up to 40% compared to 28% with bacteriophages alone.13 However, bacteriophages are specific to only one strain of bacteria, which makes them less effective for rapid treatment of polymicrobial or unidentified infections. To achieve clinical efficacy in such cases, one must combine different strains of phages. Additionally, phages do not survive without a bacterial host and must be adequately delivered to the site of infection to be efficacious.
Biodegradable polymeric coatings enhance biocompatibility and prevent biofilm formation on steel implants, offering superior mechanical strength compared to pure polymer implants. Recent research explores silica-incorporated nanoparticles for their ability to modulate drug release rates and moldability into desired shapes. While numerous alternative osteomyelitis treatment methods have been investigated, many innovative techniques are still progressing through preclinical stages before clinical trials.16
Numerous therapies and delivery systems for treating osteomyelitis show promise in animal models but await clinical validation. Key concerns include biofilms, small colony variants, infections in immunocompromised patients, such as those with diabetes, antimicrobial resistance, and concurrent infection-fracture treatments. Successful therapeutic approaches discussed include surgical debridement, antibiotics, bacteriophages, antimicrobial peptides, enzymes, quorum-sensing inhibitors, and metals. Optimizing DEMs’ effectiveness requires ongoing research on delivery systems such as hydrogels, cements, micro/nanoparticles, coatings/films, scaffolds, and sponges. Given bacterial resistance trends, exploring alternative infection control methods remains crucial.13
In Conclusion
DEMs represent a significant advancement in the management of chronic wounds and diabetic foot infections. By providing a localized and sustained release of therapeutic agents, these materials can enhance treatment efficacy, therefore achieving infection clearance, reducing the need for additional procedures and improving overall patient outcomes. With new advancements in research, drug-eluting biocomposites are poised to have an increasing role in wound care and complex infection management.
Barry I. Rosenblum, DPM, FACFAS is an Assistant Clinical Professor of Surgery at Harvard Medical School and associate chief of the division of Podiatry at Beth Israel Deaconess Medical Center in Boston, MA.
Rahul Mishra, DPM, is a CLINICAL FELLOW at Harvard Medical School and Chief Resident of the podiatric surgery residency program at Beth Israel Deaconess Medical Center.
Michelle Kung, DPM, is a Clinical Fellow at Harvard Medical School and second-year resident at Beth Israel Deaconess Medical Center.
References
1. Hobizal KB, Wukich DK. Diabetic foot infections: current concept review. Diabet Foot Ankle. 2012. 3(1). doi: 10.3402/dfa.v3i0.18409
2. Melamed EA, Peled E. Antibiotic impregnated cement spacer for salvage of diabetic osteomyelitis. Foot Ankle Int. 2012;33(3):213–9. doi:10.3113/FAI.2012.0213
3. Costerton JW, Stewart PS, Greenberg EP. Bacterial biofilms: A common cause of persistent infections. Science. 1999. 284(5418):1318-1322. doi:10.1126/science.284.5418.1318
4. Wolcott RD, Rhoads DD. A study of biofilm-based wound management in subjects with critical limb ischaemia. J Wound Care. 2008. 17(4):145-155. doi:10.12968/jowc.2008.17.4.28835
5. Frykberg RG, Banks J. Challenges in the treatment of chronic Wounds. Adv Wound Care (New Rochelle). 2015. 4(9):560-582. doi:10.1089/wound.2015.0635
6. Lipsky BA, Berendt AR, Cornia PB, et al. 2012 Infectious Diseases Society of America clinical practice guideline for the diagnosis and treatment of diabetic foot infections. Clin Infect Dis. 2012;54(12):e132-e173. doi:10.1093/cid/cis346
7. Hetrick EM, Schoenfisch MH. Reducing implant-related infections: active release strategies. Chem Soc Rev. 2006;35(9):780-789. doi:10.1039/b515219b
8. Oliver RA, Lovric V, Christou C, Walsh WR. Comparative osteoconductivity of bone void fillers with antibiotics in a critical size bone defect model [published correction appears in J Mater Sci Mater Med. 2020. 31(9):80. doi:10.1007/s10856-020-06418-1
9. Dai J, Zhou Y, Mei S. Application of antibiotic bone cement in the treatment of infected diabetic foot ulcers in type 2 diabetes. BMC Musculoskelet Disord. 2023. 24(135). https://doi.org/10.1186/s12891-023-06244-w
10. Morley R, Rothwell M, Stephenson J, McIlvenny L, Webb F, Barber A. Complex foot infections treated with surgical debridement and antibiotic loaded calcium sulfate—a retrospective cohort study of 137 cases. J Foot Ankle Surg. 2022. 61(2):239-247. doi:10.1053/j.jfas.2021.07.014
11. Gauland C. Managing lower-extremity osteomyelitis locally with surgical debridement and synthetic calcium sulfate antibiotic tablets. Adv Skin Wound Care. 2011. 24(11):515-523. doi:10.1097/01.ASW.0000407647.12832.6c
12. Elmarsafi T, Oliver NG, Steinberg JS, Evans KK, Attinger CE, Kim PJ. Long-term outcomes of permanent cement spacers in the infected foot. J Foot Ankle Surg. 2017. 56(2): 287-290. doi:10.1053/j.jfas.2016.10.022
13. Cobb LH, McCabe EM, Priddy LB. Therapeutics and delivery vehicles for local treatment of osteomyelitis. J Orthop Res. 2020;38(10):2091-2103. doi:10.1002/jor.24689
14. Boyle K, Sosa B, Osagie L, Turajane K, Bostrom MPH, Yang X. Vancomycin-laden calcium phosphate-calcium sulfate composite allows bone formation in a rat infection model. PLoS ONE. 14(9):e0222034. https://doi.org/10.1371/journal.pone.0222034
15. Ding X, Yuan Y, Lu H, et al. Analysis of the effect of antibiotic bone cement in the treatment of diabetic foot ulcer through tibia transverse transport. Orthop Surg. 2022. 14(9):2141-2149. doi:10.1111/os.13412
16. Smith M, Roberts M, Al-Kassas R. Implantable drug delivery systems for the treatment of osteomyelitis. Drug Dev Ind Pharm. 2022. 48(10):511-527. doi:10.1080/03639045.2022.2135729