The Edge: The Oxyhemoglobin Dissociation Curve, Part 2
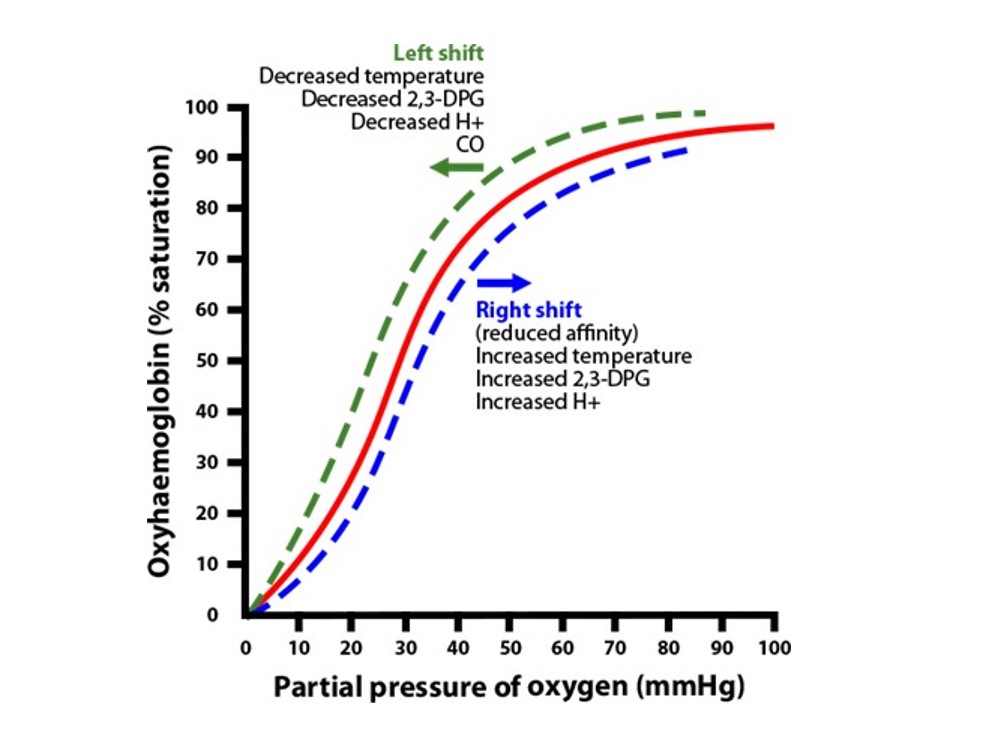
The Edge is a monthly blog series developed by EMS World and FlightBridgeED that features top EMS leaders exploring the intricacies of critical care in EMS practice. In this installment FlightBridgeED President and CEO Eric Bauer, MBA, EMT-P, completes his discussion of oxyhemoglobin.
Find the first part of this column here.
Now that we’ve learned how the oxyhemoglobin dissociation curve reflects the body’s efforts to maintain homeostasis, let’s evaluate how acute illness can change hemoglobin’s affinity for oxygen and how that might impact our patients and care.
Why is a right shift of the curve significant? Most of our patients will fall under this category, so it is essential to understand its impact. Because of the low affinity for bound oxygen this shift demonstrates, the hemoglobin (Hgb) saturation (SpO2) will be lower than normal, but the partial pressure of arterial oxygen (PaO2) will be higher. Because of this low affinity for oxygen bound to Hgb, the affinity for CO2 to bind with Hgb increases.
The raised temperature should remind you that any patient suffering from hyperthermia, fever, thyroid storm, or drug intoxications that increase body temperature will have a right shift. The raised acid levels are due to the increased CO2 and H+ ion levels that lead to acidosis. That means any patient in respiratory or metabolic acidosis should be thought of as having a right shift. The raised 2,3-DPG can manifest from a high global tissue hypoxia, causing the cells to release 2,3-DPG.
Reviewing this concept from earlier, the 2,3-DPG enters the red blood cells and binds with any deoxyhemoglobin. Once bound it causes a low-affinity state and decreases the hemoglobin’s ability to have bound oxygen. This causes oxygen to instead move freely to the bloodstream, raising the PaO2 and increasing potential delivery to the tissues.
Another element of 2,3-DPG to highlight is its impact on women who are pregnant. We know circulating blood volume increases significantly, with a large increase seen in plasma and red blood cells, by the time an expecting mom reaches her second trimester. As such, pregnant women have high concentrations of red blood cells and 2,3-DPG, so they can deliver large amounts of oxygen to the placenta and fetus. Others with potentially high levels of 2,3-DPG may include athletes who train at high altitudes to increase their RBC counts and people who live at higher altitudes.
In the end patients with a right shift on the oxyhemoglobin curve will have a lower-than-normal SaO2 and SpO2 and a higher-than-normal PaO2. This means they will have higher-than-normal releases of O2 stores to the tissues. It also means you will see lower SpO2 readings. This does not mean your patients are as hypoxic as they may appear based on the SpO2 reading. SpO2 is only an indication of the Hgb saturation and nothing more.
With that thought process, right-shift patients are not always getting oxygen molecules to the tissues either. Even though they have high PaO2 levels, this does not mean their shock state is allowing oxygen diffusion through to the tissues. Think about sepsis, for example. If there is a diffusion shunt (an inability for oxygen to move across a cell membrane from high concentration to low)—or because of interstitial fluid shifting blocking access to the tissues—the patient’s high PaO2 means nothing. If your Hgb releases its stores, then your SpO2 will fall. However, this does not represent the amount of O2 actually being delivered to the tissues and organs.
In contrast, left shifts represent a higher affinity for O2 bound to Hgb and won’t let the O2 be released from the Hgb to hypoxic tissues. This gives you a falsely high SpO2 and less oxygen being released, causing decreased tissue oxygenation (PaO2). Remember, your SpO2 reflects the amount of O2 bound to Hgb. If your Hgb is holding on to all the O2 stores and not releasing them, you will have highly saturated Hgb but low PaO2 and a corresponding potential for poor tissue oxygenation.
These patients are very sick. Basically, you will see this in low temperature, with patients suffering from hypothermia, a thyroid disorder called myxedema coma, and significant blood loss. Low acid (low CO2 and H+) causes respiratory or metabolic alkalosis disorders. Low 2,3-DPG is related to massive transfusions of packed red blood cells (PRBCs).
Trauma and Transfusion
One of the biggest teaching points regarding the left shift is seen in trauma and massive transfusion guidelines. When looking at trauma treatment guidelines, we have learned that permissive hypotension is key, and maintaining a mean arterial pressure of 60–65 mmHg in a non-head-injury patient will be sufficient to perfuse the brain and kidneys. In addition, massive transfusion protocols (MTPs) are now being employed. It is not uncommon to see patients receive 6–10 units of PRBCs in a 24-hour period. We have learned the most optimal ratio is a 1:1:1 combination of fresh, frozen plasma, platelets, and PRBCs.
One of the biggest issues with massive transfusion is that the PRBCs are stored in citrate. The citrate encapsulates the 2,3-DPG molecules found in the cells and binds with calcium found in the blood. Due to this, patients who are bleeding out may receive blood that has nonfunctional 2,3-DPG. Remember, when tissue hypoxia ensues the cells release 2,3-DPG to ultimately bind with deoxyhemoglobin. Because of the citrate, the 2,3-DPG can’t facilitate that binding, causing the low-affinity state which leads to the increased release of oxygen to the tissues.
It will take as long as 8 hours after PRBC administration until 2,3-DPG becomes unencapsulated and useful, with a full 24 hours until all the 2,3-DPG becomes unencapsulated. This process can be acceleerated by the administration of calcium chloride or calcium gluconate during blood administration, especially if MTPs are employed. It is important to fully resuscitate these patients with good oxygenation strategies and potentially even intubation with mechanical ventilation if this is the best way to optimize oxygen delivery.
Lastly, low PaO2 is the result of the Hgb being highly bound with oxygen and the high-affinity state, resulting in poor unloading of oxygen available for tissue oxygenation. Remember, the SpO2 may show a false normal due to the left-shift phenomenon. Such patients may be having tissue hypoxia instead.
Case Conclusion
Which patient from last month’s opening scenario do you treat first? The correct answer is to treat your patient. As we stated then, it is not wrong to treat the patient with the SpO2 of 88%; it is wrong to think the patient with the SpO2 of 96% is perfectly stable and not in need of oxygen therapy. Because this patient could be suffering from an acute disease process related to the left shift, the SpO2 could be simply a misleading reflection of the highly oxygen-bound Hgb.
Does this translate to good tissue oxygenation in a patient who is tissue-hypoxic? No. As we highlighted with this simple scenario, we must understand our patients fully before we make decisions within our treatment guidelines. A thorough assessment is essential. Remember, SpO2 is only as good as your understating of the big picture.
Eric Bauer, MBA, FP-C, CCP-C, C-NPT, EMT-P, is the president and CEO of FlightBridgeED, LLC. He has worked in the EMS field for 30 years, spending the past 19 in the HEMS industry. Eric is an internationally recognized best-selling author, speaker, and educator and holds a Bachelor of Business in Health Care Administration and a Master of Business Administration. He was the recipient of the 2018 John Jordan Award for Excellence in Transport Medicine Journalism by the Air & Surface Transport Nurses Association.