Therapeutic Modalities of Human β Defensin-2 with Prospective Significance in Diabetic Wound Treatment
Abstract
Background: Diabetic Wounds (DW) are a debilitating complication of diabetes. Although various therapeutic strategies are available for DW management, none of them meet all the fundamentals due to the multifaceted pathophysiology of DW. Given the ever-present threat of DW, novel improved therapeutic strategies and the fortification of DW research deserve better prioritization. Purpose: The current review aims to provide the detailed role of human β defensin-2 (HBD-2) in skin wounds with a prospective significance in DW treatment. Methods: This review was conducted through searches of relevant abstracts, research and review articles, and patents, in Scopus, Web of Science, PubMed, Google Scholar, and Clinical Trials databases of English language articles. Results: HBD-2 is a critical innate immune-response protein that defends the human body from infections. Recent reports also support the role of HBD-2 in suppressing inflammation and promoting proliferation and angiogenesis. Based on the literature, its therapeutic administration has been proposed to maintain the equilibrium of systemic homeostasis in DW. Conclusion: It has been hypothesized that HBD-2 could be a crucial modulator for controlling chronic inflammation and size by promoting proliferation and angiogenesis, opening up new therapy options in DW.
Introduction
Diabetic Wound (DW) is a late-stage type 2 diabetes complication that emanates from interplay amongst inflammation, vasculopathy, neuropathy, and impaired tissue regeneration, all on the background of insulin resistance.1,2 Although various pharmacological approaches are available, the management of DW is still in its infancy.3 The DW treatment puzzle is complex because optimization of glycaemic control has little or no effect on the course of disease progression at later stages of DW.4 Hence, the unique etiopathogenic connotation between diabetes and DW becomes less relevant at later phases of disease progression. This theoretical problem highlights the necessity of developing a novel strategy focused on targeting the molecular modalities of DW.
DWs are majorly divided into 3 types on a clinical basis: ischemic, neuropathic, and neuro-ischemic.5 Though the pathogenesis of DW remains incomprehensible, prolonged inflammation and infection are considered to be crucial factors that are hindering the subsequent phases in the course of DW healing.6 Further, the conceptual consideration of the DW treatment strategy depends on the pathogenetic variables, such as matrix metalloproteinase-9 and chemokines7, which indirectly affect tissue regeneration. However, only a few variables fit the comprehensible rationale for the pharmacological management of DW.
HBD-2 and its 3D-crystal structure
Human β defensin-2 (HBD-2) is a cationic antimicrobial peptide (AMP), discovered in 19978, which is a part of innate and adaptive immunity. It comprises 41 amino acids with a gene of 4kb, expressed mainly in the skin, epithelium, and mucous. HBD-2 consists of 6 cysteine residues at positions 1-5, 2-4, and 3-6, united by 3 disulfide bridges. The secondary structure of HBD-2 reveals the presence of an α-helix, and 3 β-sheets organized in an antiparallel mode in the N-terminal and C-terminal regions.9-11 The primary folding of the peptide is possible by the disulfide bonds between the α-helix and first β-sheet, which possess a domain of Gly-X-CysIV. Though the active site is present in the N-terminal region, the antimicrobial activity of the peptide is mainly caused by the presence of cationic residues present in the C-terminal region of the peptide.12,13 The remaining biological properties of the peptide are dependent on the specific conformation of the N-terminal region of the HBD-2. Further, its amphipathic nature and the presence of disulfide bridges are responsible for resistance to degradation by proteases.14,15
Genetic information, regulation, and expression of HBD-2
Genetically, the gene encoding HBD-2 is located on the 8p23 chromosome and composed of 2 exons separated by intron and 5’-3’ non-translatable region. The primary exon has 81 bp and encodes a signal peptide, whereas the secondary exon has 238 bp encoding an anionic peptide (propeptide) and mature peptide.16-18 The 5’ region of HBD-2 possesses specific sites for binding transcription factors such as nuclear factor kappa light chain enhancer of activated B cells (NF-kB), CCAAT/enhancer-binding proteins (C/EBP), and nuclear factor interleukin-6 (NF-IL-6), which are necessary for their expression.18-20 In addition, HBD-2 has a polymorphic nature, and the gene copies differ in each individual. Several studies reported that 2 to 12 copies are present per diploid genome, and the number varies according to expression level. Further, these variations may affect the immune system function.20,21
HBD-2 is usually produced as a functionally inactive peptide called prepro-defensin, and to attain its activity, HBD-2 must undergo a post-translational modification process and form a mature peptide. The inactive peptide comprises a mature peptide, propeptide, and hydrophobic pre-peptide signal. Further, the inactive peptide is cleaved by proteases in the Golgi apparatus to release mature peptide on the surface of the epithelium.22,23
Regulation of HBD-2 expression in the human body involves multiple signaling pathways; bacterial proteins (LPS) induce HBD-2 expression through transcription factors (NF-kB, myocyte enhancement factor [MEF], NF-IL-6, and activator protein [AP1-3])24-27 and signaling pathways (mitogen-activated protein kinase [MAPKs], phosphoinositide 3-kinase [PI3K] and protein kinase C [PKC]).28-31 Toll-like receptors induce HBD-2 expression by initiating intracellular signaling pathways (activation of NF-KB promotes the gene expression of various proinflammatory mediators).
The chemotactic property of HBD-2 induces its production by interacting with the chemokine receptor-6 (CCR6) receptors32 and also depends on vitamin D and protseas-coupled receptors (PARs).33-36
Recently, HBD-2 has been considered a multifunctional modulator with antimicrobial and immunomodulatory properties. The ability of HBD-2 to bind to the various ligands suggests that there might be more signaling pathways and targets still to be discovered, which may have unique pharmacological activities.
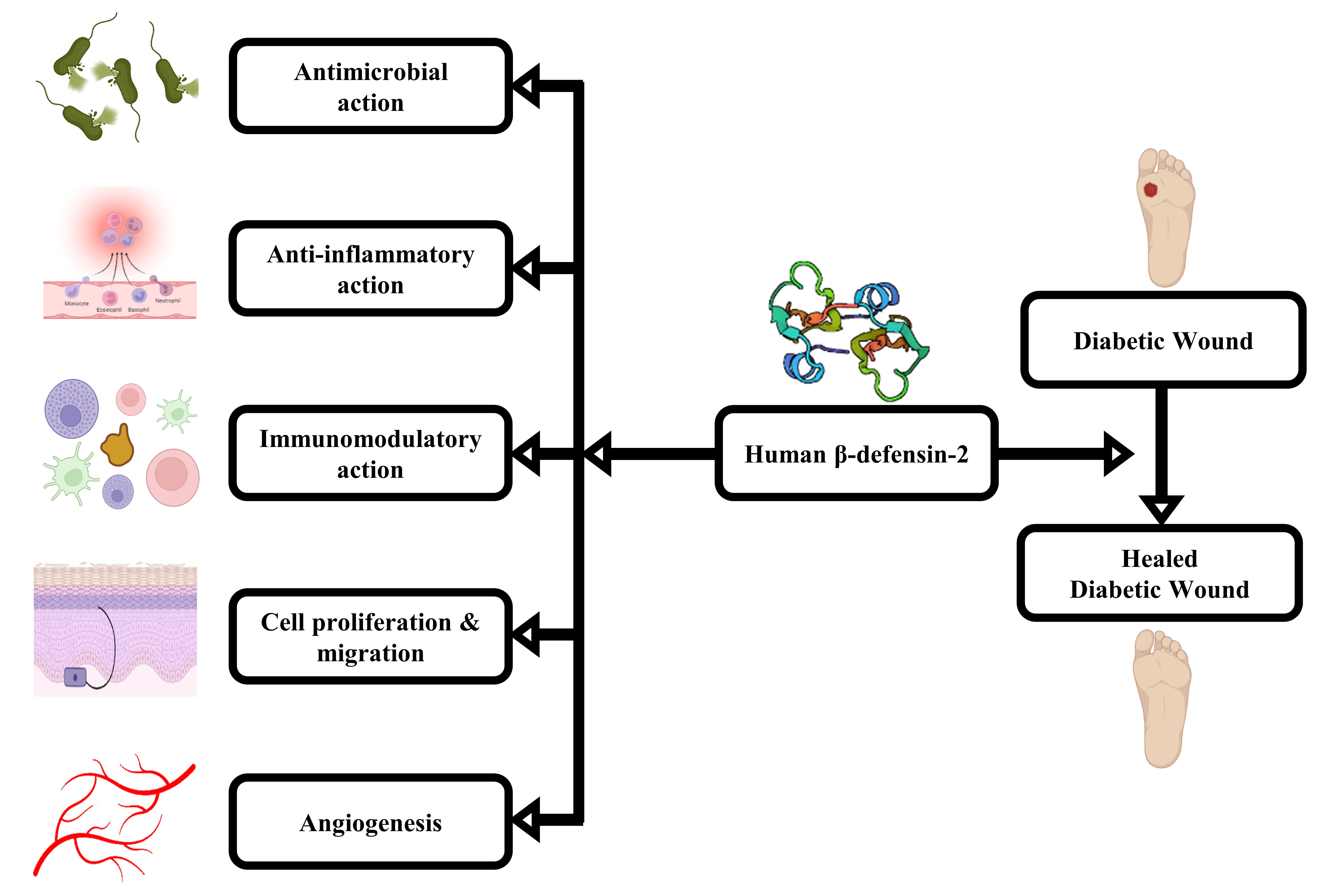
In this paper, we detailed the role of HBD-2 as a pharmacological agent in treating DW (Figure 1). This evidence-based estimation emanates from the ubiquitous properties of HBD-2 to modulate inflammatory responses, micro- and macro-circulation, tissue regeneration, and infection control.
Methods
This review was accomplished by conducting searches of relevant abstracts, research and review articles, and patents in Scopus, Web of Science, PubMed, Google Scholar, and Clinical Trials databases of all English language articles. Diabetes, diabetic foot ulcers, diabetic wound, diabetic foot, diabetic foot infections, wound management, antimicrobial peptides, and human beta defensin-2 were some of the search terms used.
Discussion
HBD-2 as an antimicrobial agent
One of the important strategies in the management of DW is infection control. Clinically, DW infections are defined by ≥2 classic findings of purulence or inflammation and classified as severe, moderate, or mild. The common pathogenic bacteria in DW infections are S. aureus, S. epidermis, E. coli, K. pneumonia, P. aeruginosa, and Proteus sp. Most DW infections are considered polymicrobial, which suggests the use of a specific drug with a broad spectrum of activity in managing DW infections.
Bacterial resistance to conventional antibiotics is a major life-threatening concern and the main reason for extensive research to design or develop novel therapeutics. Though microorganisms developed various ways to escape AMPs, their resistance mechanism only depends on the direct killing of bacteria.37 One of the preferred strategies in combating resistance is the combination of AMPs with conventional antibiotics.38
To better understand AMPs, it is essential to evaluate their kinetics in local and systemic infection and inflammation. In general, HBD-2 has become the best choice of therapy for regulating and controlling infection.
In such circumstances, the external administration of HBD-2 in treating DW infections might accelerate the healing of DW. The unique properties of HBD-2, such as a being broad-spectrum, an endogenous peptide, non-toxic to eukaryotic cells, and less prone to resistance, indicate its usage would be more beneficial in tackling DW infections. A study by Milner et al clearly depicts that low levels of HBD-2 are responsible for a higher risk of wound infection and sepsis, which indicates that exogenous administration of HBD-2 might reduce the infection risk in DW patients.39
Mechanistically, the negatively charged components of bacterial membranes serve as targets for HBD-2. Lipopolysaccharide (LPS) and teichoic acid are targets for gram-negative and gram-positive bacteria, respectively. HBD-2 is tremendously effective against gram-negative bacteria (E. coli, P. aeruginosa, S. marcescens, and A. baumannii) and the fungus C. albicans. However, it possesses only bacteriostatic properties against gram-positive bacteria (S. aureus). The electrostatic interaction between the positively charged peptide and negatively charged bacterial membrane displaces the bacteria’s lipids to form pores, eventually leading to cell lysis and death of the bacteria (Figure 2a).40,41
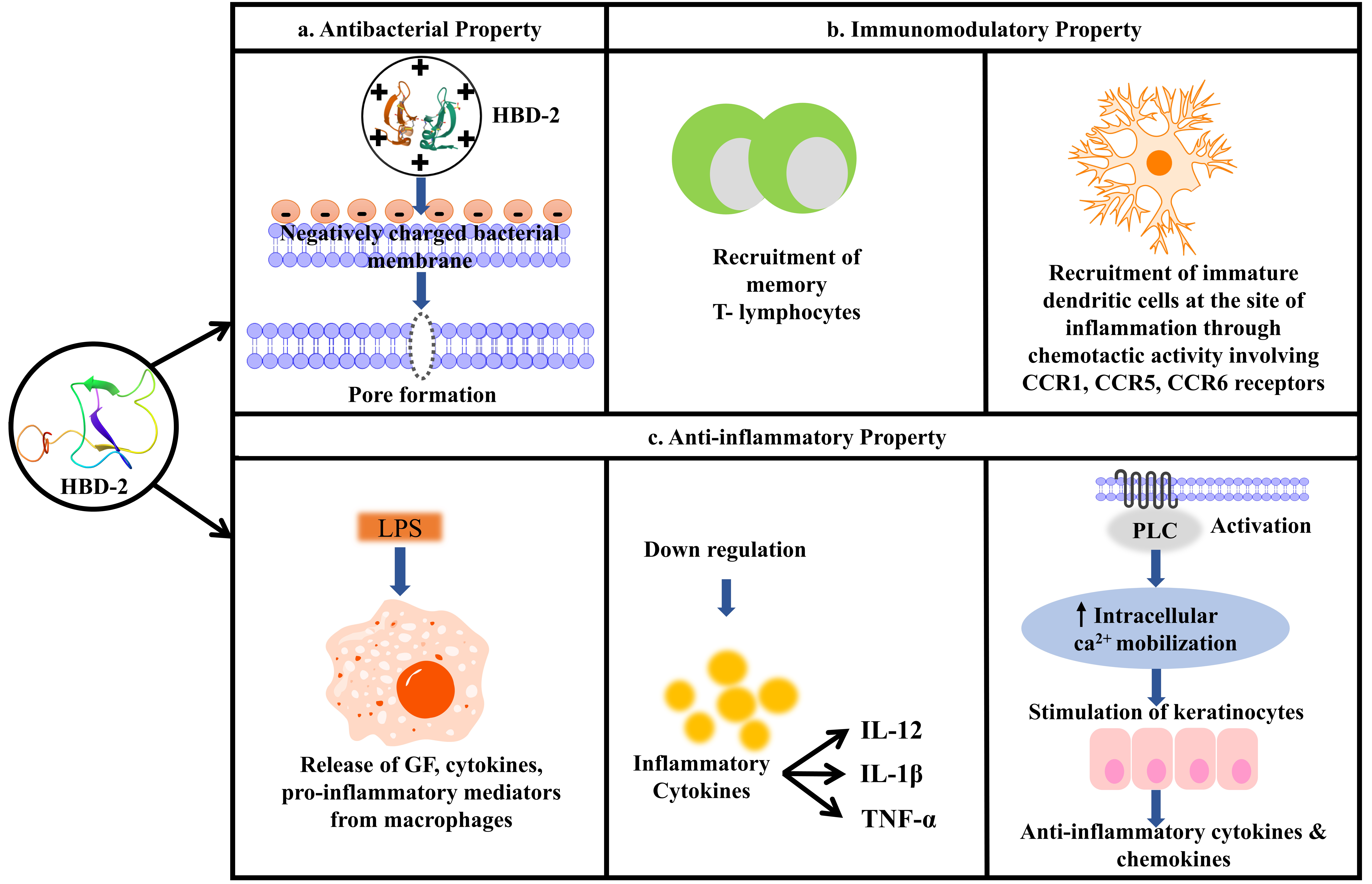
From the literature, it is clear that infections caused by wild or resistant strains can be counteracted by administering HBD-2, motivating its use for DW healing.
HBD-2 as an immunomodulator agent
One of the major pathogenetic variables for impaired healing of DW is the macrophage, that exhibit different immunological functions in the skin. Tissue macrophages are usually derived from monocytes that migrate through the vessel wall and release the enzyme that cleaves extracellular matrix (ECM) proteins, thus forming a pore for monocytes to migrate to the DW bed. Tissue macrophages are activated typically by LPS or interferon-γ and, alternatively, by IL-4 and IL-13.42,43 Further, macrophages can synthesize and secrete various cytokines, growth factors, and proinflammatory mediators, including nitric oxide (NO), IL-6, and tumor necrosis factor-a (TNF-α). In addition, IL-4-activated macrophages play a crucial role in angiogenesis, collagen synthesis, and fibrosis.44 Because of these properties, macrophages/monocytes are considered a suitable model for evaluating the immunomodulatory activity of novel therapeutics.
HBD-2 plays a crucial role in innate and adaptive immunity and constitutes the first line of host defense against microbial infections. Several mechanisms have been reported for the adaptive immunity of HBD-2: (1) recruitment of memory T-lymphocytes, effector cells of adaptive immunity; (2) recruitment of immature dendritic cells from circulating blood to the site of inflammation by chemotactic activity involving CCR1, CCR5, and CCR6 receptors (Figure 2b); and (3) the presentation of dendritic cells by forming defensin-antigen complexes.45,46 As evident from the previously published reports, HBD-2 exhibits an immunomodulatory effect. Koeninger et al reported that recombinant HBD-2 suppressed dendritic cell-mediated secretion of inflammatory cytokines such as IL-1β, IL-12, and TNF-α, which was dependent on CCR2 signaling and decreased NF-κB, but increased cAMP-response element binding protein (CREB) phosphorylation. In general, innate immunity mainly involves the complement system that is composed of 30 proteins of human serum and is activated by 3 pathways: classical, alternative, and lectin. HBD-2 was also found to inhibit this classic complement pathway by binding to C1q of the complement system and regulating uncontrolled activation of the complement system as evidenced by Bhat et al.47 Later, Niyonsaba et al reported HBD-2 as a mast cell chemotaxin via a phospholipase-C-dependent pathway.48 The study by Boniotto et al clearly exemplified the role of HBD-2 in the innate and adaptive immune systems. HBD-2 stimulated cytokines and chemokines and upregulated more selectively in peripheral blood mononuclear cell (PBMC).49
Thus, owing to its contribution to innate and adaptive immune responses, it can solely be used as an agent in controlling inflammation, which is a long-term and crucial phase of wound healing.
HBD-2 as an anti-inflammatory agent
Non-healing chronic wounds or DWs are most prevalent and fail to heal through normal phases of wound healing and remain in a chronic inflammatory state. Both underlying local stimulus and systemic defects might contribute to the delay of inflammatory phase in DW healing, thereby creating a cascade of responses that intensify the hostile environment. One of the most systemic defects affecting the inflammatory phase of the normal wound healing process is pyoderma gangrenosum, which can only be treated by immune suppression. Further, a venous disease that enhances and perpetuates inflammation is usually due to intrinsic factors such as increased hydrostatic pressure and hyperglycemia. Bacterial components, foreign substances of necrotic tissue, and hypoxia are other powerful local stimuli capable of releasing macrophages and neutrophils in a controlled manner. Moreover, bacterial components might contribute to impaired healing mechanisms by attenuating the inflammation or interfering with cell-matrix interactions.
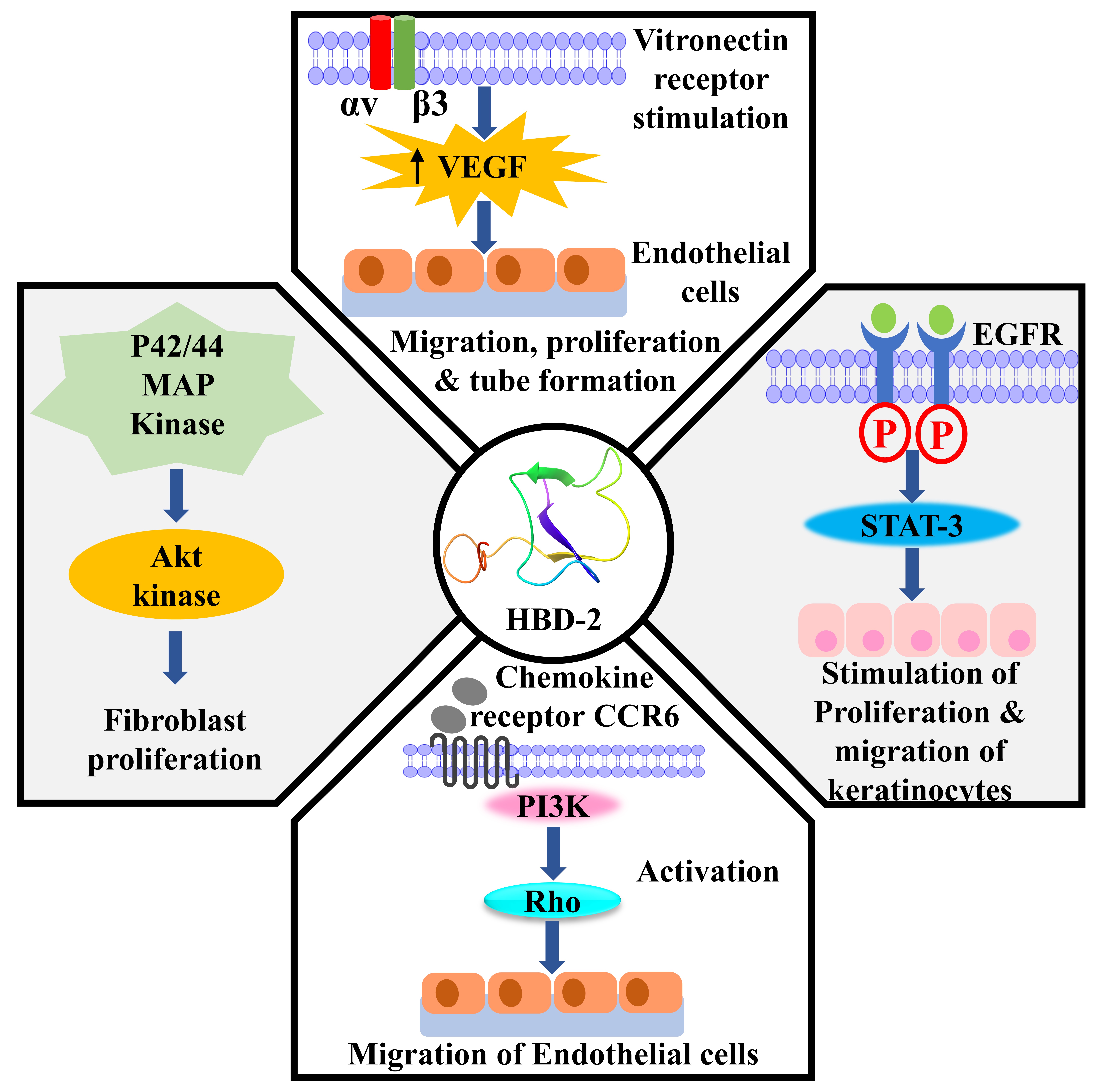
Sanchez et al produced a recombinant HBD-2 and studied its anti-inflammatory properties. The study depicts that HBD-2 treatment in LPS-activated PBMC and upregulated anti-inflammatory cytokines IL-10 and IL-24 while decreasing the levels of proinflammatory TNFα, IL-1β, and IL-23 (Figure 2c).⁵⁰ Later, Pinkerton et al reported the therapeutic application of HBD-2 significantly suppressed the influx of inflammatory mediators such as cytokines IL-9 and IL-13 into bronchoalveolar lavage fluid.51
There is growing evidence that HBD-2 treatment affects multiple pathways associated with immunity and infections. Among the key features of immunomodulating and anti-inflammatory activity is the downregulation of inflammatory cytokines like IL-1β, IL-12, and TNF-α. This literature suggests that HBD-2 may selectively inhibit the vicious cycle of inflammatory disbalance in DW, thereby alleviating the intensity of inflammation.
HBD-2 as cell-proliferating, migrating, and angiogenic agent
The acute wound healing cascade is well synchronized by inflammatory cells, cytokines, MMPs, growth factors (GFs), endothelial cells, keratinocytes, and fibroblasts. GFs are active polypeptides involved in all the phases of wound healing. Chronic wounds frequently exhibit defects in the type and levels of GFs due to alteration of their expression, diminished synthesis and release, trapping, and excessive degradation. ECM synthesis is characterized by the balance between its formation and degradation; ECM is regulated by the following growth factors: vascular endothelial (VEGF), insulin-like (IGF-I, IGF-II), transforming (TGF-β), keratinocyte (KGF), platelet-derived (PDGF), epidermal (EGF), fibroblast (FGF), and TNF-α and IL-6, which are noticeably decreased in the high-glucose environment. GFs also play a crucial role in initiating and sustaining the phases of wound healing. Any modification, down-regulation, and rapid degradation of GF leads to delayed healing of DW.
HBD-2 plays a pivotal role in promoting the expression of growth factors, cell proliferation, and migration as evidenced by several reports. Winter et al, 2011 reported that HBD-2 exhibited significant proliferation of squamous cells. The molecular mechanism is still unclear and requires further investigation.52 A study by Baroni et al exemplifies the role of HBD-2 in the proliferation, migration, and tube formation of umbilical vein endothelial cells. HBD-2 increased endogenous secretion of VEGF by stimulating αvβ3 vitronectin receptor which, in turn, stimulated migration, proliferation, and tube formation of endothelial cells.53 Bobin et al demonstrated the role of designer HBD-2 (A-HBD-2) in wound healing by stimulating the proliferation and migration of keratinocytes via epidermal growth factor receptor (EGFR) and signal transducer activator and transmission 3 (STAT3) phosphorylation. In addition, HBD-2 provoked intracellular Ca2+ mobilization and stimulated keratinocytes to produce pro- and anti-inflammatory cytokines and chemokines via phospholipase C activation.54 Another study by Li et al exemplified that HBD-2 induced human conjunctival fibroblast proliferation via activation of Akt kinase and p42/p44 MAP kinase in a dose-dependent fashion.55 From the study by Vongsa et al it is evident that HBD-2 activated Rho and PI3K and mobilized Ca2+ through chemokine receptor CCR6 to evoke actin cytoskeleton reorganization of colonic epithelial cells, which eventually contributes to efficient epithelial migration and mucosal barrier repair.56 Otte et al reported that HBD-2 enhanced intestinal wound healing and was also involved in stimulating the migration of intestinal epithelial cells.57 From the shreds mentioned above, HBD-2 can act as a cell proliferation, migration, and angiogenesis promoter and explicitly participate in tissue regeneration in DW (Figure 3).
Conclusion
DW is the leading complication resulting in organ amputation and affecting the quality of life. Hence, dire attention is required in DW research to develop novel therapeutic strategies. In this paper, we exemplified the multifunctional modalities of HBD-2, an endogenous peptide with prospective significance in managing DW. From the above pieces of evidences, it is anticipated that the internal stimulation or external application of HBD-2 might benefit DW management. Moreover, detailed investigations are highly warranted to understand the exact mechanism of HBD-2 in the DW treatment. In summary, HBD-2 can likely be used as a potential therapeutic agent to accelerate the healing of DW.
Author Affiliations
1Department of Pharmaceutical Chemistry, NIMS Institute of Pharmacy, NIMS University, Jaipur, Rajasthan-303121, India
2Department of Pharmacology, NIMS Institute of Pharmacy, NIMS University, Jaipur, Rajasthan-303121, India
3Department of Pharmacology, Faculty of Pharmacy, Mohan Babu University, Tirupati, Andhra Padesh-517102, India
References
1. Prentki M, Nolan CJ. Islet beta cell failure in type 2 diabetes. J Clin Invest. 2006;116(7):1802-1812. doi:10.1172/JCI29103
2. Anselmo MI, Nery M, Parisi MC. The effectiveness of educational practice in diabetic foot: a view from Brazil. Diabetol Metab Syndr. 2010;2(1):45. Published 2010 Jun 29. doi:10.1186/1758-5996-2-45
3. Palamarchuk VI, Turaiev PI, Muz' MI, Shuliarenko OV, Hordiienko BO. Treatment of pateints with diabetic foot syndrome. Klin Khir. 2010;(1):59-60.
4. Jirkovská A. Stĕzejní otázky terapie syndromu diabetické nohy [Basic questions in therapy of the diabetic foot]. Vnitr Lek. 2002;48(6):542-548.
5. Quattrini C, Jeziorska M, Malik RA. Small fiber neuropathy in diabetes: clinical consequence and assessment. Int J Low Extrem Wounds. 2004;3(1):16-21. doi:10.1177/1534734603262483
6. Boulton AJ. The diabetic foot: grand overview, epidemiology and pathogenesis. Diabetes Metab Res Rev. 2008;24 Suppl 1:S3-S6. doi:10.1002/dmrr.833
7. Hasnan J, Yusof MI, Damitri TD, Faridah AR, Adenan AS, Norbaini TH. Relationship between apoptotic markers (Bax and Bcl-2) and biochemical markers in type 2 diabetes mellitus. Singapore Med J. 2010;51(1):50-55.
8. Harder J, Bartels J, Christophers E, Schröder JM. A peptide antibiotic from human skin. Nature. 1997;387(6636):861. doi:10.1038/43088
9. Bruhn O, Grötzinger J, Cascorbi I, Jung S. Antimicrobial peptides and proteins of the horse--insights into a well-armed organism. Vet Res. 2011;42(1):98. Published 2011 Sep 2. doi:10.1186/1297-9716-42-98
10. Corrales-Garcia L, Ortiz E, Castañeda-Delgado J, Rivas-Santiago B, Corzo G. Bacterial expression and antibiotic activities of recombinant variants of human β-defensins on pathogenic bacteria and M. tuberculosis. Protein Expr Purif. 2013;89(1):33-43. doi:10.1016/j.pep.2013.02.007
11. Machado LR, Ottolini B. An evolutionary history of defensins: a role for copy number variation in maximizing host innate and adaptive immune responses. Front Immunol. 2015;6:115. Published 2015 Mar 18. doi:10.3389/fimmu.2015.00115
12. Taylor K, Clarke DJ, McCullough B, et al. Analysis and separation of residues important for the chemoattractant and antimicrobial activities of beta-defensin 3. J Biol Chem. 2008;283(11):6631-6639. doi:10.1074/jbc.M709238200
13. Schneider JJ, Unholzer A, Schaller M, Schäfer-Korting M, Korting HC. Human defensins. J Mol Med (Berl). 2005;83(8):587-595. doi:10.1007/s00109-005-0657-1
14. Hans M, Madaan Hans V. Epithelial antimicrobial peptides: guardian of the oral cavity. Int J Pept. 2014;2014:370297. doi:10.1155/2014/370297
15. Underwood M, Bakaletz L. Innate immunity and the role of defensins in otitis media. Curr Allergy Asthma Rep. 2011;11(6):499-507. doi:10.1007/s11882-011-0223-6
16. Semple F, Dorin JR. β-Defensins: multifunctional modulators of infection, inflammation and more?. J Innate Immun. 2012;4(4):337-348. doi:10.1159/000336619
17. Chen H, Xu Z, Peng L, et al. Recent advances in the research and development of human defensins. Peptides. 2006;27(4):931-940. doi:10.1016/j.peptides.2005.08.018
18. Lan H, Chen H, Chen LC, et al. The first report of a Pelecaniformes defensin cluster: characterization of β-defensin genes in the crested ibis based on BAC libraries. Sci Rep. 2014;4:6923. Published 2014 Nov 5. doi:10.1038/srep06923
19. Tsutsumi-Ishii Y, Nagaoka I. Modulation of human beta-defensin-2 transcription in pulmonary epithelial cells by lipopolysaccharide-stimulated mononuclear phagocytes via proinflammatory cytokine production. J Immunol. 2003;170(8):4226-4236. doi:10.4049/jimmunol.170.8.4226
20. Frew L, Stock SJ. Antimicrobial peptides and pregnancy. Reproduction. 2011;141(6):725-735. doi:10.1530/REP-10-0537
21. McDermott AM. The role of antimicrobial peptides at the ocular surface. Ophthalmic Res. 2009;41(2):60-75. doi:10.1159/000187622
22. Guaní-Guerra E, Santos-Mendoza T, Lugo-Reyes SO, Terán LM. Antimicrobial peptides: general overview and clinical implications in human health and disease. Clin Immunol. 2010;135(1):1-11. doi:10.1016/j.clim.2009.12.004
23. Martin E, Ganz T, Lehrer RI. Defensins and other endogenous peptide antibiotics of vertebrates. J Leukoc Biol. 1995;58(2):128-136. doi:10.1002/jlb.58.2.128
24. Becker MN, Diamond G, Verghese MW, Randell SH. CD14-dependent lipopolysaccharide-induced beta-defensin-2 expression in human tracheobronchial epithelium. J Biol Chem. 2000;275(38):29731-29736. doi:10.1074/jbc.M000184200
25. Lu Z, Kim KA, Suico MA, Shuto T, Li JD, Kai H. MEF up-regulates human beta-defensin 2 expression in epithelial cells. FEBS Lett. 2004;561(1-3):117-121. doi:10.1016/S0014-5793(04)00138-3
26. Mineshiba J, Myokai F, Mineshiba F, Matsuura K, Nishimura F, Takashiba S. Transcriptional regulation of beta-defensin-2 by lipopolysaccharide in cultured human cervical carcinoma (HeLa) cells. FEMS Immunol Med Microbiol. 2005;45(1):37-44. doi:10.1016/j.femsim.2005.01.008
27. Harder J, Meyer-Hoffert U, Teran LM, et al. Mucoid Pseudomonas aeruginosa, TNF-alpha, and IL-1beta, but not IL-6, induce human beta-defensin-2 in respiratory epithelia. Am J Respir Cell Mol Biol. 2000;22(6):714-721. doi:10.1165/ajrcmb.22.6.4023
28. Jang BC, Lim KJ, Paik JH, et al. Up-regulation of human beta-defensin 2 by interleukin-1beta in A549 cells: involvement of PI3K, PKC, p38 MAPK, JNK, and NF-kappaB. Biochem Biophys Res Commun. 2004;320(3):1026-1033. doi:10.1016/j.bbrc.2004.06.049
29. Scharf S, Hippenstiel S, Flieger A, Suttorp N, N'Guessan PD. Induction of human β-defensin-2 in pulmonary epithelial cells by Legionella pneumophila: involvement of TLR2 and TLR5, p38 MAPK, JNK, NF-κB, and AP-1. Am J Physiol Lung Cell Mol Physiol. 2010;298(5):L687-L695. doi:10.1152/ajplung.00365.2009
30. Kim YJ, Shin HS, Lee JH, Jung YW, Kim HB, Ha UH. Pneumolysin-mediated expression of β-defensin 2 is coordinated by p38 MAP kinase-MKP1 in human airway cells. J Microbiol. 2013;51(2):194-199. doi:10.1007/s12275-013-2579-x
31. Méndez-Samperio P. Role of antimicrobial peptides in host defense against mycobacterial infections. Peptides. 2008;29(10):1836-1841. doi:10.1016/j.peptides.2008.05.024
32. Jarczak J, Kościuczuk EM, Lisowski P, et al. Defensins: natural component of human innate immunity. Hum Immunol. 2013;74(9):1069-1079. doi:10.1016/j.humimm.2013.05.008
33. Yurie H, Ikeguchi R, Aoyama T, et al. The efficacy of a scaffold-free Bio 3D conduit developed from human fibroblasts on peripheral nerve regeneration in a rat sciatic nerve model. PLoS One. 2017;12(2):e0171448. Published 2017 Feb 13. doi:10.1371/journal.pone.0171448
34. Pinheiro da Silva F, Machado MC. Antimicrobial peptides: clinical relevance and therapeutic implications. Peptides. 2012;36(2):308-314. doi:10.1016/j.peptides.2012.05.014
35. Huang FC. De Novo sphingolipid synthesis is essential for Salmonella-induced autophagy and human beta-defensin 2 expression in intestinal epithelial cells. Gut Pathog. 2016;8:5. Published 2016 Feb 18. doi:10.1186/s13099-016-0088-2
36. Chung WO, Hansen SR, Rao D, Dale BA. Protease-activated receptor signaling increases epithelial antimicrobial peptide expression. J Immunol. 2004;173(8):5165-5170. doi:10.4049/jimmunol.173.8.5165
37. Peschel A, Sahl HG. The co-evolution of host cationic antimicrobial peptides and microbial resistance. Nat Rev Microbiol. 2006;4(7):529-536. doi:10.1038/nrmicro1441
38. Giacometti A, Cirioni O, Ghiselli R, et al. Interaction of antimicrobial peptide temporin L with lipopolysaccharide in vitro and in experimental rat models of septic shock caused by gram-negative bacteria. Antimicrob Agents Chemother. 2006;50(7):2478-2486. doi:10.1128/AAC.01553-05
39. Milner SM, Ortega MR. Reduced antimicrobial peptide expression in human burn wounds. Burns. 1999;25(5):411-413. doi:10.1016/s0305-4179(98)00192-2
40. Chow BT, Soto M, Lo BL, Crosby DC, Camerini D. Antibacterial Activity of Four Human Beta-Defensins: HBD-19, HBD-23, HBD-27, and HBD-29. Polymers. 2012; 4(1):747-758. https://doi.org/10.3390/polym4010747
41. Cieślik M, Bagińska N, Górski A, Jończyk-Matysiak E. Human β-Defensin 2 and its postulated role in modulation of the immune response. Cells. 2021;10(11):2991. Published 2021 Nov 3. doi:10.3390/cells10112991
42. Geng K, Ma X, Jiang Z, et al. Innate immunity in diabetic wound healing: Focus on the mastermind hidden in chronic inflammatory. Front Pharmacol. 2021;12:653940. Published 2021 Apr 21. doi:10.3389/fphar.2021.653940
43. Mouritzen MV, Petkovic M, Qvist K, et al. Improved diabetic wound healing by LFcinB is associated with relevant changes in the skin immune response and microbiota. Mol Ther Methods Clin Dev. 2021;20:726-739. Published 2021 Feb 15. doi:10.1016/j.omtm.2021.02.008
44. Moura J, Rodrigues J, Gonçalves M, Amaral C, Lima M, Carvalho E. Impaired T-cell differentiation in diabetic foot ulceration. Cell Mol Immunol. 2017;14(9):758-769. doi:10.1038/cmi.2015.116
45. Auvynet C, Rosenstein Y. Multifunctional host defense peptides: antimicrobial peptides, the small yet big players in innate and adaptive immunity. FEBS J. 2009;276(22):6497-6508. doi:10.1111/j.1742-4658.2009.07360.x
46. Yang D, Biragyn A, Kwak LW, Oppenheim JJ. Mammalian defensins in immunity: more than just microbicidal. Trends Immunol. 2002;23(6):291-296. doi:10.1016/s1471-4906(02)02246-9
47. Bhat S, Song YH, Lawyer C, Milner SM. Modulation of the complement system by human beta-defensin 2. J Burns Wounds. 2007;5:e10. Published 2007 Jan 10.
48. Niyonsaba F, Iwabuchi K, Matsuda H, Ogawa H, Nagaoka I. Epithelial cell-derived human beta-defensin-2 acts as a chemotaxin for mast cells through a pertussis toxin-sensitive and phospholipase C-dependent pathway. Int Immunol. 2002;14(4):421-426. doi:10.1093/intimm/14.4.421
49. Boniotto M, Jordan WJ, Eskdale J, et al. Human beta-defensin 2 induces a vigorous cytokine response in peripheral blood mononuclear cells. Antimicrob Agents Chemother. 2006;50(4):1433-1441. doi:10.1128/AAC.50.4.1433-1441.2006
50. Mailänder-Sánchez D, Kjaerulf S, Sidelmann Brinch K, et al. DOP083 Recombinant subcutaneous human beta-Defensin 2 (hBD2) ameliorates experimental colitis in different in vivo models. J Crohns Colitis. 2017;11(suppl_1):S75-S76
51. Pinkerton JW, Kim RY, Koeninger L, et al. Human β-defensin-2 suppresses key features of asthma in murine models of allergic airways disease. Clin Exp Allergy. 2021;51(1):120-131. doi:10.1111/cea.13766
52. Winter J, Pantelis A, Reich R, et al. Human beta-defensin-1, -2, and -3 exhibit opposite effects on oral squamous cell carcinoma cell proliferation. Cancer Invest. 2011;29(3):196-201. doi:10.3109/07357907.2010.543210
53. Baroni A, Donnarumma G, Paoletti I, et al. Antimicrobial human beta-defensin-2 stimulates migration, proliferation and tube formation of human umbilical vein endothelial cells. Peptides. 2009;30(2):267-272. doi:10.1016/j.peptides.2008.11.001
54. Mi B, Liu J, Liu Y, et al. The designer antimicrobial peptide A-hBD-2 facilitates skin wound healing by stimulating keratinocyte migration and proliferation. Cell Physiol Biochem. 2018;51(2):647-663. doi:10.1159/000495320
55. Li J, Raghunath M, Tan D, Lareu RR, Chen Z, Beuerman RW. Defensins HNP1 and HBD2 stimulation of wound-associated responses in human conjunctival fibroblasts. Invest Ophthalmol Vis Sci. 2006;47(9):3811-3819. doi:10.1167/iovs.05-1360
56. Vongsa RA, Zimmerman NP, Dwinell MB. CCR6 regulation of the actin cytoskeleton orchestrates human beta defensin-2- and CCL20-mediated restitution of colonic epithelial cells. J Biol Chem. 2009;284(15):10034-10045. doi:10.1074/jbc.M805289200
57. Otte JM, Werner I, Brand S, et al. Human beta defensin 2 promotes intestinal wound healing in vitro. J Cell Biochem. 2008;104(6):2286-2297. doi:10.1002/jcb.21787