ADVERTISEMENT
No-Fluoroscopy Crossing of Chronic Total Occlusions Using Ocelot Optical Coherence Tomography Guided Catheter
Abstract
Purpose: To demonstrate a case series where the use of optical coherence tomography (OCT) greatly reduced or eliminated fluoroscopy during the crossing of peripheral arterial chronic total occlusions (CTOs) when using the Ocelot catheter (Avinger). Methods: Fifteen patients were treated for peripheral arterial CTOs between January 2013 and June 2013. Sixteen lesions were attempted and crossed via the true lumen under OCT guidance using the Ocelot Catheter. Eleven males and 4 females were treated, with a mean patient age of 73 (50-91) years. Time measurements were recorded for diagnostic angiography, CTO crossing fluoroscopy, and therapeutic fluoroscopy times. Results: 100% of CTOs in this series were crossed successfully via the true lumen without the use of assist or re-entry devices. Mean CTO crossing fluoroscopy time was 0.24 (14.4 seconds) ± 0.36 minutes using the Ocelot. In comparison, mean diagnostic and therapeutic fluoroscopic times were 5.99±3.07 minutes and 14.88±6.36 minutes, respectively. Mean lesion length treated was 18.6 cm (18.63±8.58 cm). All 16 lesions were reduced from 100% stenosis to less than 10% stenosis. The treated lesions represented a spectrum of calcification (mild/none=2; moderate=8; severe=6) The mean contrast volume administered across all procedures was 176.66±50.08 mL (median=180; range 100-230 mL). There were no adverse events reported at 9-month follow-up. Conclusion: The Ocelot catheter allows for safe, quick, and efficient crossing of peripheral arterial CTOs while eliminating, or significantly reducing, fluoroscopic exposure and associated contrast administration.
VASCULAR DISEASE MANAGEMENT 2015;12(12):E230-E241
Key words: chronic occlusion, peripheral vascular disease, superficial femoral artery, atherosclerosis, vascular disease
__________________________________________________________
Current technologies used for crossing peripheral arterial chronic total occlusions (CTOs) rely solely on angiographic imaging for positioning and guidance. While several techniques, devices, and guidewires have been developed and refined for use in CTOs, the inability of angiography to adequately visualize occluded arterial segments makes intervention in this setting technically challenging.1
Angioscopy, intravascular ultrasound (IVUS), and optical coherence tomography (OCT) are applications that may allow an operator to cross total occlusions with the aid of intra-arterial imaging. Optical coherence tomography is the first fiber-optic technology that has successfully been integrated within an FDA-approved CTO crossing catheter. The Ocelot catheter system (Avinger) utilizes OCT at the distal tip of the catheter to understand orientation to arterial structures while advancing spiral-fluted wedges through CTOs. This lumivascular approach provides visibility across vessel luminal and wall structures, allowing physicians to guide, orient, and preserve true luminal CTO crossings. Recent studies demonstrate excellent safety and efficacy of lumivascular therapy.2 Lumivascular is differentiated from endovascular procedures that rely solely on angiographic guidance.
Angiography’s two-dimensional projection format and the lack of soft tissue contrast enable external tracking of catheters and wires. Likewise, x-ray fluoroscopy is unable to accurately represent vessel tortuosity or deep wall pathology. Additional limitations include safety concerns over excessive X-ray exposure (>40 min fluoroscopy time) and contrast loads averaging 350 mL.1,3,4
Fluoroscopy has been a valuable tool to interventionalists, however exposure to radiation poses a burden to the physician, nursing staff, and patient. Radiation exposure can lead to cataracts, cancer, and abnormal hematologic counts.5,6 It is estimated that for every 100,000 catheter-based cardiac procedures, 83 will result in new cancer diagnoses.7 Techniques are employed to help lessen the exposure amounts to some degree, but prolonged exposure time can have a negative impact, especially to parts of the body that are unprotected during procedures such as the eyes, hands, and thyroid gland.8 Eyes are especially sensitive to high doses of ionizing radiation. In a study led by Ciraj-Bjelac et al, 52% of interventional cardiologists (n=56) showed radiation-associated posterior lens opacities compared with 9% in the control group (n=22).9,10
Angiographic imaging and fluoroscopy with iodinated contrast remain the gold standard for imaging patients with vascular disease.11 Fluoroscopy is correlated with contrast loading for arterial mapping. High volumes of contrast can predispose patients to contrast-induced nephropathy (CIN), which is a cause of acute renal failure (ARF) and can worsen pre-existing renal function impairment in patients undergoing diagnostic imaging studies.10,12 Use of iodinated radiocontrast agents has increased, especially in the field of cardiology, and is associated with a significant increase in morbidity, mortality, prolonged hospital stays, and, as a consequence, increased health care costs.13,14
In addition to the proven safety and efficacy of the Ocelot catheter when treating peripheral arterial CTOs, this case series demonstrates the added benefits of reducing fluoroscopic burden during lesion crossing. Fifteen patients were evaluated as part of a prospective, nonrandomized case series conducted at St. John Hospital (Detroit, Michigan) demonstrating significantly reduced or eliminated fluoroscopy use for peripheral arterial CTO crossing.
Materials and Methods
OCT-Guided Catheter
The Ocelot catheter is 110 cm in length, it incorporates a 155 mm optical fiber for OCT functionality, and it is compatible with 6 Fr sheaths and 0.014˝ guidewires. Spiral wedges located on the tip of the catheter advance the catheter across the CTO by rotating in both clockwise and counterclockwise directions.
Patient Selection and Treatment
All patients were selected based on the angiographic diagnosis of chronic total occlusion. Exemplary angiography prior and post treatment is shown in Figure 1. Patients were appropriately consented for surgery and anesthesia prior to intervention. Fifteen successive patients diagnosed with CTOs (11 males, 4 females) were treated on an all-comer basis using the Ocelot catheter between January 2013 and June 2013. Mean patient age was 73 years (50-91) and mean lesion length was 18.6 cm (18.6±8.67 cm; range 4-30 cm). Patient demographics and lesion characteristics are summarized in Table 1.
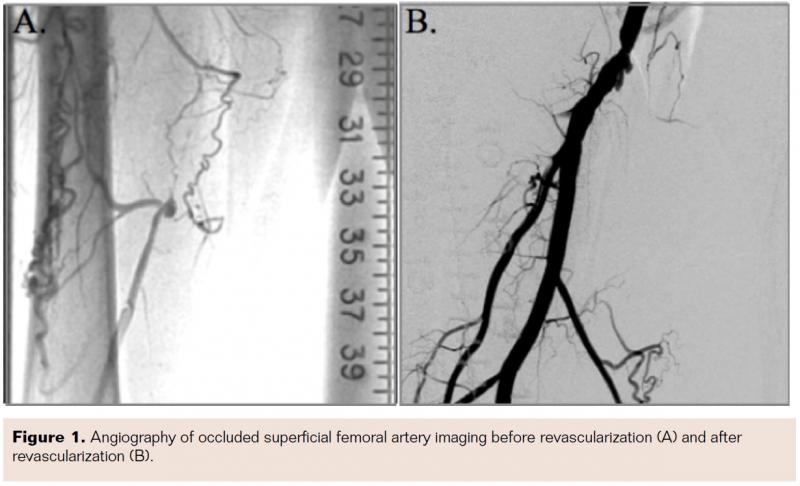
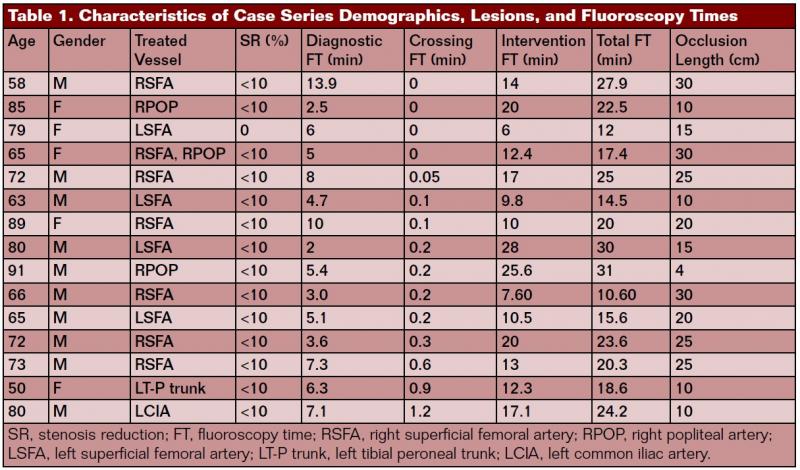
CTO Treatment With Ocelot OCT-Guided Catheter
In all cases, contralateral iliofemoral artery access (7 right femoral access, 8 left femoral access) was obtained using the modified Seldinger technique. The Ocelot was introduced over an 0.014˝ wire and advanced through the CTO using OCT guidance per the instructions for use (IFU). CTO crossing was confirmed via both OCT image and distal wire passage in the true lumen. Post crossing therapies varied based on lesion characteristics. Patients were monitored during the procedure and postoperatively at 30- and 60-day intervals.
CTO Treatment/Crossing Fluoroscopic Measurements
Measured fluoroscopic values were standardized to the following: diagnostic fluoroscopy was measured from the point of arterial access until the introduction of the Ocelot catheter; CTO crossing fluoroscopy was measured from engagement of the proximal cap to the crossing of the distal cap of the CTO; therapeutic fluoroscopy measured any intervention subsequent to CTO crossing; and total fluoroscopy was the sum of all measured values.
Results
CTO Crossing
Sixteen CTOs in 15 patients (100%) were crossed successfully using a standalone Ocelot catheter. The OCT-guided intraluminal crossing was performed within the luminal to medial boundaries of the artery (within the true lumen), without the need for subintimal tracking and without requiring ancillary crossing wires or other support catheters. The crossing precision of the studied OCT lesions was enabled due to the targeted mode of Ocelot catheter progression: the Ocelot catheter is advanced through the fibrotic or stenotic CTO occlusions based on the rotation of spiral wedges located on the tip of the catheter, in both clockwise and counterclockwise directions (Figure 2). The OCT radiopaque directional markers (Figures 2 and 3) allow for the placement of the distal tip within the occlusive CTO tissue, opposite and away from the healthy vascular lumen boundary (layered tissue, Figure 4). In this cohort, no dissections or perforations were noted. The therapeutic recanalization of the crossed lesions was then completed using a combination of therapies, including angioplasty and/or atherectomy, as preferred by the operator and based on the clinical indication. Notably, because of the intraluminal recanalization, no stent placement was required.
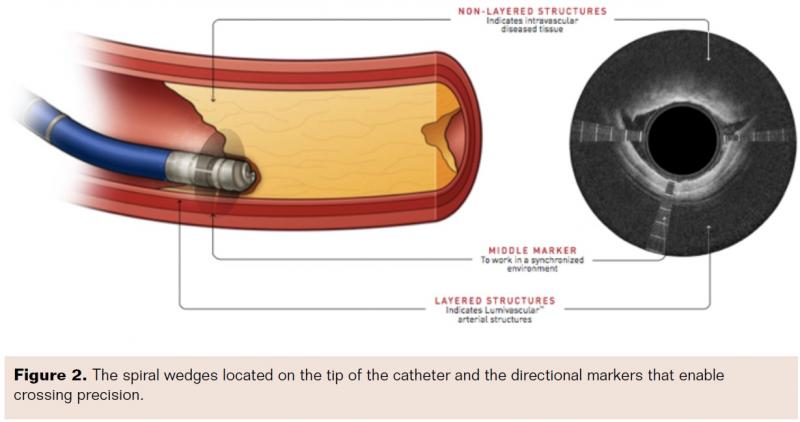
CTO Crossing Times
Because the Ocelot catheter position and advancement is entirely based on its real-time OCT imaging, we postulated that it could effectively eliminate the need for fluoroscopic imaging during the CTO crossings. Thus, we monitored the minimal fluoroscopy time necessary to cross the studied lesions. For 5/16 lesions, which measured 21.25±8.93 cm in length, no fluoroscopy (0 min) was required during crossing; for 8/16 lesions, which measured 18.63±8.58 cm in length, ≤30 seconds of fluoroscopy (10.25±4.79 seconds) were required during crossing; for 2/16 lesions, which measured 18.4 ± 8.37cm in length, ≤60 seconds of fluoroscopy (mean = 45 seconds) were required during crossing; and for 1/16 lesions, which measured 10 cm, >60 seconds (72 seconds) of fluoroscopy were required during crossing. The use of limited fluoroscopy appeared to be independent of vessel calcification grade. In mild/noncalcified lesions, the average CTO crossing fluoroscopy time was 0.05 minutes; with moderately severe calcium, 0.31 minutes; and with severe calcium, 0.24 minutes.
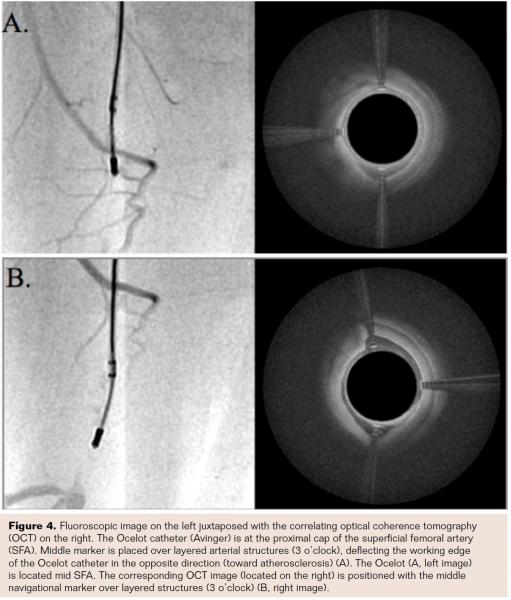
Mean diagnostic fluoroscopy time across all lesions (n=16) was 5.99±3.07 minutes, mean crossing fluoroscopy time was 0.24±0.36 minutes, mean intervention fluoroscopy time was 14.88±6.36 minutes, and the mean procedural (total) fluoroscopy time was 20.88±6.24 minutes (Figure 5). In 16/16 lesions the mean contrast volume administered across procedures was 176.66±50.08 mL. Postprocedure stenosis reduction in 15/15 patients was measured from 100% to <10% stenosis.
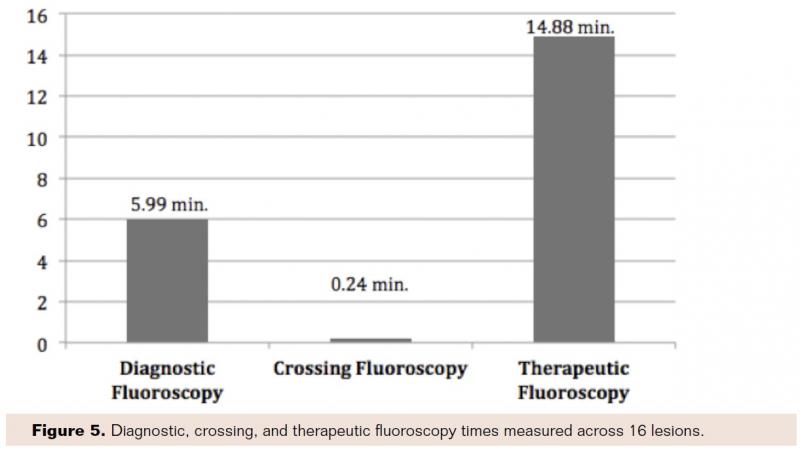
Case Example: Low-Fluoroscopy CTO Crossing Using the Ocelot Catheter
A representative low-fluoroscopy CTO case is shown in Figure 6. A 68-year-old female presented with multiple risk factors for cardiovascular disease and PVD, including history of smoking, hypertension, hyperlipidemia, diabetes mellitus, history of myocardial infarction, and ischemic cardiomyopathy (ejection fraction 35%). The patient presented with intermittent claudication for several years and rest pain over the last 6 months, Rutherford Class IV, with no critical limb ischemia and with ankle brachial index of 0.5 on the right leg. Angiogram of the right lower extremity showed complete occlusion of the left distal SFA to P3 popliteal segment (Figure 6). Further complicating the matter, the patient had a prosthetic knee replacement, which blinded A/P x-ray viewing of the occluded segment. The 10 cm 100% CTO lesion was crossed stand-alone using the OCT guided Ocelot catheter. The total crossing time was 2 minutes and 17 seconds. CTO crossing fluoroscopy was measured at 12 seconds (0.2 mins) and included angiographic confirmation of distal wire advancement following distal cap crossing (Figure 7). The OCT imagery during the crossing highlighted a true lumen recanalization with eccentric disease burden composed of mostly fibrin rich de novo plaque and a moderate calcium nodule (Figure 8). The lesion was then treated with angioplasty (Chocolate balloon; Cordis Corporation). Residual, stand-alone post-therapy stenosis was measured at <10%. Importantly, OCT was able to reliably guide a true lumen crossing in the no-stent popliteal zone even with prosthetic hardware blocking angiographic visualization of the artery.
Discussion
This paper reports on a prospective, nonrandomized, single-center case series, where the Ocelot catheter was safely and effectively used to cross a combination of 16 superficial femoral and popliteal arterial CTOs across 15 patients. Of the CTO lesions treated, 100% were successfully crossed using a stand-alone Ocelot catheter, without dissection, perforation, or the need for ancillary crossing wires.
Moreover, by using a lumivascular approach, the operator was able to drastically reduce and, in some cases, eliminate the use of fluoroscopy while crossing the CTO. Radiation exposure is an occupational health concern for interventionalists who are chronically exposed to ionizing radiation. Numerous publications have demonstrated the potential hazards of accumulated radiation exposure for cataracts and oncogenesis including glioblastoma multiforme, meningiomas, thyroid cancer,15 and many others.16-30 Segal et al conducted a retrospective study and assessed radiation exposure for 346 procedures at a single center. They concluded that radiation exposure during lower-extremity vascular procedures is substantial and should be considered during treatment planning.31
In an acute setting, reduction of contrast burden associated with long fluoroscopic runs may alleviate any adverse impact patients predisposed to renal insufficiency may encounter. These would include electrolyte imbalances, congestive heart failure exacerbation, pulmonary edema, dialysis, and worsened adjusted mortality.32 Additionally, OCT would maintain superior image quality compared to other renal protective imaging mediums, such as CO2.10
Discussion around fluoroscopic reduction for lower-extremity interventions has been ongoing. Various studies have examined how different x-ray systems, catheterization protocols, and correct use of radiation protection devices can affect radiation exposure of cardiologists.33 Most of these solutions have been reactive (increased external protection, proximity to radiation source, etc.) as opposed to proactive for fluoroscopic reduction. Lumivascular tools are an adjuvant to the standard endovascular approach of fluoroscopic-only imaging during intervention. Through utilization of a lumivascular approach, physicians can concentrate on the OCT images for safe and successful CTO crossings while enjoying the benefits of a reduction in potentially toxic radiation exposure.
In this lumivascular case series, we appreciate significant and consistent reduction in CTO crossing fluoroscopy times when OCT is available as an adjuvant imaging modality. Importantly, it appears that even severe calcium may be limited to low fluoroscopic burdens. Lumivascular catheters may provide a clinical benefit to the current endovascular standard of care where severely calcified vessels demand increased crossing times, fluoroscopic burdens, and contrast loads.
Comparison of the Ocelot catheter to standard-of-care (endovascular) CTO crossing times highlights the dramatic ability to reduce fluoroscopy for CTO crossing (Table 2). Charalambous et al treated 26 lesions using the Frontrunner XP (Cordis Corporation) catheter. CTO crossing fluoroscopy was measured at a mean of approximately 8 minutes. This cohort was similar to our reported lesion population with mean length of 17.6 cm. The Frontrunner XP demonstrated an 88.12% crossing efficacy.34 Gandini et al evaluated the Crosser catheter (C.R. Bard) in 12 patients with 75% efficacy crossing CTOs (median length 26 cm) and a mean fluoroscopic crossing time of 4.05 minutes. Likewise, Staniloae et al report on a series of CTO lesions (n=73) treated using the Crosser catheter. Subset analysis of the SFA/popliteal lesions demonstrates 64% efficacy (n=36) crossing CTOs (mean length=14.3-20.7 cm). Mean crossing fluoroscopy time was 14±10 minutes.35
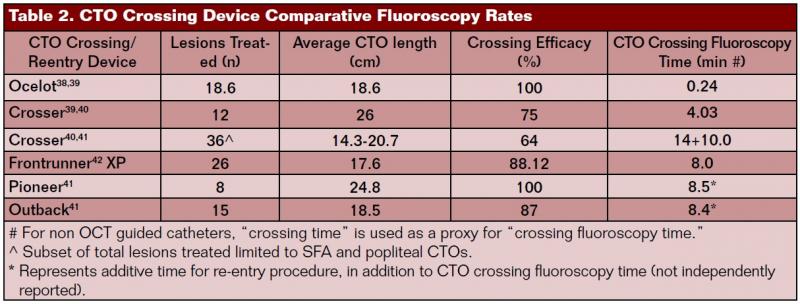
When true luminal crossing is not successful, re-entry catheters add time, cost, and fluoroscopic burden. In 23 SFA lesions with an average length of 18.5-24.8 cm, Smith et al demonstrated an average added fluoroscopic time of 8.4 and 8.5 minutes for the Outback LTD (Cordis Corporation) and Pioneer Plus PPLUS 120 (Medtronic) catheters, respectively.36
With regard to downstream treatment following successful CTO crossing, OCT-guided therapeutic catheters (i.e. atherectomy) may provide similar benefits through reduced fluoroscopic exposure and contrast administration. By minimizing the reliance on fluoroscopy for both CTO crossing and therapeutic intervention, it may be possible to significantly reduce x-ray radiation for physicians, the cath lab staff, and patients. Additionally, contrast reduction may prove valuable in the treatment of patients prone to renal insufficiency.
Conclusion
To date, the lack of advanced imaging on endovascular catheters has perpetuated the reliance on angiography to determine catheter location and position during advancement through a CTO. The advent of OCT guidance enables the operator to appreciate many arterial factors not visualized by fluoroscopy: intravessel morphology, transition points between healthy arterial structures and atherosclerosis, masked aneurysms, arterial branch orientation, subintimal vs true luminal positioning, and native vessel reconstitution with resulting active blood flow.37 These imaging advancements have allowed for continued improvement in the safety and efficacy of SFA CTO crossings.2
In this single site case series, we demonstrate that either zero or minimal fluoroscopy is needed when crossing CTOs with the Ocelot catheter system. In this cohort of patients, fluoroscopic time was greatly reduced or eliminated during CTO crossing, regardless of varying lesion locations, length, calcification grade, and multivessel involvement.
Reducing fluoroscopy is a reproducible feature of lumivascular applications. Further research is needed to determine the overall benefits to ancillary health care providers, physicians, and patients. Future lumivascular applications utilizing OCT guidance for peripheral and coronary therapies may add additional benefits through improved outcomes and reduced fluoroscopic burden during both diagnostic and therapeutic interventions.
Editor’s note: The authors have completed and returned the ICMJE Form for Disclosure of Potential Conflicts of Interest. Dr. Davis reports consultancy, honoraria, stock or stock options, and travel reimbursement from Avinger.
Manuscript received July 17, 2015; manuscript accepted August 10, 2015.
Address for correspondence: Thomas P. Davis, MD, Saint John Hospital and Medical Center, Department of Internal Medicine, Section of Cardiology, Detroit, MI 48236, United States. Email: tpdavis60@aol.com
References
- Courtney BK, Munce MR, Anderson, KJ, et al. Innovations in imaging for chronic total occlusions: a glimpse into the future of angiography’s blind spot. Eur Heart J. 2008;29(5);583-593.
- Schwindt A, Reimers B, Scheinert D, et al. Crossing chronic total occlusions with the Ocelot system: the initial European experience. EuroIntervention. 2013;9(7):854-862.
- Buller CE, Dzavik V, Carere RG, et al. Primary stenting versus balloon angioplasty in occluded coronary arteries: The total occlusion study of Canada (TOSCA). Circulation. 1999;100(3):236-242.
- Suzuki S, Furui S, Kohtake H, et al. Radiation exposure to patients skin during percutaneous coronary intervention for various lesions, including chronic total occlusion. Circ J. 2006;70(1):44-48.
- Brilakis ES, Patel VG. What you can’t see can hurt you! J Invasive Cardiol. 2012;24(9):421.
- Buchanan GL, Chieffo A, Mehill J, et al. The occupational effects of interventional cardiology: results from the WIN for safety survey. EuroIntervention. 2012;8(6):658-663.
- Segal E, Weinberg I, Leichter I, Klimov, Giri J, Bloom A. Patient radiation exposure during percutaneous endovascularization of the lower extremity. J Vasc Surg. 2013;58(6):1556-1562.
- Kesavachandran CN, Haamann F, Nienhaus A. Radiation exposure and adverse health effects of interventional cardiology staff. Rev Environ Contam Toxicol. 2013;222:73-91.
- Ciraj-Bjelac O, Rehani MM, Sim KH, Liew HB, Vano E, Kleiman NJ. Risk for radiation-induced cataract for staff in interventional cardiology: is there reason for concern? Catheter Cardiovasc Interv. 2010;76(6):826-834.
- Jacob S, Michel M, Spaulding C, et al. Occupational cataracts and lens opacities in interventional cardiology (O’CLOC study): are x-rays involved? Radiation induced cataracts and lens opacities. BMC Public Health. 2010;10:537.
- Dowling K, Kan H, Siskin G, et al. Safety of limited supplemental iodinated contrast administration in azotemic patients undergoing CO2 angiography. J Endovasc Ther. 2003;10(2):312-316.
- Alessandri N, Lanzi L, Garante CM, et al. Prevention of acute renal failure post-contrast imaging in cardiology: a randomized study. Eur Rev Med Pharmacol Sci. 2013;17(Suppl 1):13-21.
- Nash K, Hafeez A, Hou S. Hospital-acquired renal insufficiency. Am J Kidney Dis. 2002;39(5):930-936.
- Rihal CS, Textor SC, Grill DE, et al. Incidence and prognostic importance of acute renal failure after percutaneous coronary intervention. Circulation. 2002;105(19):2259-2264.
- Sinnot B, Ron E, Schneider AB. Exposing the thyroid to radiation: a review of its current extent, risks and implications. Endocr Rev. 2010;31(5):756-773.
- Roguin A, Goldstein J, Bar O, Goldstein JA. Brain and neck tumors among physicians performing interventional procedures. J Am Coll Cardiol. 2013;111(9):1368-1372.
- Jansen M, Yip S, Louis DN. Molecular pathology in adult gliomas: diagnostic, prognostic, and predictive markers. Lancet Neurol. 2010;9(7):717-726.
- Inskip PD, Linet MS, Heineman EF. Etiology of brain tumors in adults. Epidemiol Rev. 1995;17(2):382-414.
- Blettner M, Schlehofer B, Samkange-Zeeb F, Berg G, Schlaefer K, Schüz J. Medical exposure to ionising radiation and the risk of brain tumours: Interphone study group. Germany Eur J Cancer. 2007;43(13):1990-1998.
- Loomis DP, Savitz DA. Mortality from brain cancer and leukaemia among electrical workers. Br J Ind Med. 1990;47(9):633-638.
- Grayson JK. Radiation exposure, socioeconomic status, and brain tumor risk in the US Air Force: a nested case-control study. Am J Epidemiol. 1996;143(5):480-486.
- Goldstein JA, Balter S, Cowley M, et al; Interventional Committee of the Society of Cardiovascular Interventions. Occupational hazards of interventional cardiologists: prevalence of orthopedic health problems in contemporary practice. Catheter Cardiovasc Interv. 2004;63(4):407-411.
- Ciraj-Bjelac O, Rehani MM, Sim KH, Liew HB, Vano E, Kleiman NJ. Risk for radiation induced cataract for staff in interventional cardiology: is there reason for concern? Catheter Cardiovasc Interv. 2010;76(6):826-834.
- Venneri L, Rossi F, Botto N, et al. Cancer risk from professional exposure in staff working in cardiac catheterization laboratory: insights from the National Research Council’s Biological Effects of Ionizing Radiation VII Report. Am Heart J. 2009;157(1):118-124.
- Matanoski GM, Seltser R, Sartwell PE, Diamond EL, Elliott EA. The current mortality rates of radiologists and other physician specialists: specific causes of death. Am J Epidemiol. 1975;101(3):199-210.
- Ait-Ali L, Andreassi MG, Foffa I, Spadoni I, Vano E, Picano E. Cumulative patient effective dose and acute radiation-induced chromosomal DNA damage in children with congenital heart disease. Heart. 2010;96(4):269-274.
- Renaud L. A 5-y follow-up of the radiation exposure to in-room personnel during cardiac catheterization. Health Phys. 1992:62(1):10-15.
- Finkelstein MM. Is brain cancer an occupational disease of cardiologists? Can J Cardiol. 1998;14(11):1385-1388.
- Vañó E1, González L, Guibelalde E, Fernández JM, Ten JI. Radiation exposure to medical staff in interventional and cardiac radiology. Br J Radiol. 1998;71(849):954-960.
- Hardell L, Mild KH, Påhlson A, Hallquiest A. Ionizing radiation, cellular telephones and the risk for brain tumours. Eur J Cancer Prev. 2001;10(6):523-529.
- Segal E, Weinberg I, Leichter I, Klimov A, Giri J, Bloom AI. Patient radiation exposure during percutaneous endovascular revascularization of the lower extremity. J Vasc Surg. 2013;58(6):1556-1562.
- Finn WF. The clinical and renal consequences of contrast-induced nephropathy. Nephrol Dial Transplant. 2006;21(6):i2-i10.
- Kuon E, Birkel J, Schmitt M, Dahm JB. Radiation exposure benefit of a lead cap in invasive cardiology. Heart. 2003;89(10):1205-1210.
- Charalambous N, Schäfer PJ, Trentmann J, et al. Percutaneous intraluminal recanalization of long, chronic superficial femoral and popliteal occlusions using the Frontrunner XP CTO device: a single-center experience. Cardiovasc Intervent Radiol. 2010;33(1):25-33.
- Staniloae CS, Mody KP, Yadav SS, Han SY, Korabathina R. Endoluminal treatment of peripheral chronic total occlusions using the Crosser® recanalization catheter. J Invasive Cardiol. 2011;23(9):359-362.
- Smith M, Pappy R, Hennebry TA. Re-entry devices in the treatment of peripheral chronic occlusions. Tex Heart Inst J. 2011;38(4):392-397.
- Tearney GJ1, Regar E, Akasaka T, et al. Consensus standards for acquisition, measurement, and reporting of intravascular optical coherence tomography studies: a report from the International Working Group for Intravascular Optical Coherence Tomography Standardization and Validation. J Am Coll Cardiol. 2012;59(12):1058-1072.
- Davis T. Lumivascular approach to crossing chronic total occlusions. J Am Coll Cardiol. 2014;64:(11S) Supplement B: B157-158.
- Liang GZ, Zhang FX. Novel devices and specialized techniques in recanalization of peripheral arterychronic total occlusions (CTOs)—a literature review. Int J Cardiol. 2013;165(3):423-429.
- Laird J, Joye J, Sachdev N, et al. Recanalization of infrainguinal chronic total occlusions with the crosser system: results of the PATRIOT trial. J Invasive Cardiol. 2014;26(10):497-504.
- Jacobs DL, Motaganahalli L, Cox DE, Wittgen CM, Peterson GJ. True lumen re-entry devices facilitate subintimal angioplasty and stenting of total chronic occlusions: initial report. J Vasc Surg. 2006;43(6):1291-1296.
- Shetty R, Vivek G, Thakkar A, Prasad R, Pai U, Nayak K. Safety and efficacy of the frontrunner XP catheter for recanalization of chronic total occlusion of the femoropopliteal arteries. J Invasive Cardiol. 2013:25(7):344-347.