Transcatheter Aortic Valve-in-Valve Implantation in Failed Stentless Valves: A Single-Center Experience
© 2025 HMP Global. All Rights Reserved.
Any views and opinions expressed are those of the author(s) and/or participants and do not necessarily reflect the views, policy, or position of the Journal of Invasive Cardiology or HMP Global, their employees, and affiliates.
J INVASIVE CARDIOL 2025. doi:10.25270/jic/25.00006. Epub February 20, 2025.
Abstract
Objectives. Valve-in-valve transcatheter aortic valve implantation (ViV-TAVI) offers an alternative to repeat cardiac surgery in selected patients. However, ViV-TAVI in previously implanted stentless valves is more challenging than in a stented valve. This single-center study aimed to analyze the outcomes of ViV-TAVI in stentless valves.
Methods. Data were retrospectively collected from 1476 patients undergoing TAVI between January 2018 and December 2023. The primary study endpoint was 30-day and follow-up mortality. Secondary outcomes included Valve Academic Research Consortium (VARC)-3 technical success, VARC-3 device success, and VARC-3 early safety.
Results. A total of 15 patients underwent ViV-TAVI within previously implanted stentless valves. The mean age of the patients was 78.1 ± 9.3 years, with a median STS-PROM score of 4.30 (3.05, 6.75). The mean time between SAVR and TAVI was 14.7 ± 3.9 years. Eight (53.3%) patients presented with severe aortic regurgitation, while 5 (33.3%) patients had severe aortic stenosis. There were no surgical conversions, but a single case of transcatheter heart valve malposition required the deployment of a second valve. One patient died within 30 days. VARC-3 technical success was achieved in 87.6% (n = 13) of patients, device success in 80% (n = 12), and early safety in 73.3% (n = 11). The median follow-up period was 2.9 (range, 1.3-4.3) years, during which 4 patients died.
Conclusions. Careful patient selection is critical for ViV-TAVI procedures because of the high risk of complications, which is increased with stentless valves in addition to the standard risks associated with the ViV procedure. Larger studies are warranted to confirm these findings.
Introduction
In recent years, biological valves have been used for surgical aortic valve replacement (SAVR) far more frequently than mechanical valves.1 This shift is partly driven by the aging population, which has led to a greater number of older patients requiring aortic valve replacement. Additionally, the age threshold for implanting biological valves has been progressively lowered, contributing to their increased use.2 Currently, the most frequent type of bioprostheses employed for SAVR are stented valves, with only a minority of patients receiving stentless valves. Stentless valves can be effectively employed in the surgical management of endocarditis, aortic aneurysms, aortic dissections, and deteriorated aortic valves.3,4 These alternatives provide optimal hemodynamics and enhance left ventricular function, while eliminating the need for lifelong anticoagulation and preventing patient-prosthesis mismatch.5,6
The growing use of stentless valves over the past decades has resulted in an increase in the number of reoperations for these valves, a trend expected to continue because all bioprosthetic valves suffer from structural valve deterioration (SVD) over time with comparable failure rates between stented and stentless valves.7 The bioprosthetic tissue deteriorates at a rate estimated between 3.4% at 5 years and up to 50% by 8 years.8 Indeed, stentless valves also suffer from long-term durability concerns, as they often require reoperation within 7 to 15 years.9-11 These valves are prone to extensive calcification over time, which can complicate surgical interventions, increasing the need for complex aortic root reconstructions.12
In this context, valve-in-valve (ViV) transcatheter aortic valve implantation (TAVI) has emerged as a less invasive alternative to redo surgery for selected high-risk patients. However, ViV-TAVI in stentless valves is technically more challenging than stented valves because of the absence of fluoroscopic markers, the variability of root anatomy, the lack of a stented frame for valve anchoring, and higher rates of aortic regurgitation rather than aortic stenosis.12 These challenges lead to a higher rate of complications, including device malposition, paravalvular leak (PVL), and coronary obstruction.13 For this purpose, this study sought to describe the outcomes of ViV-TAVI procedures in stentless valves from a single-center experience.
Methods
This study included patients who underwent ViV-TAVI due to SVD of a previously implanted stentless valve at the Lankenau Heart Institute in Wynnewood, Pennsylvania, between January 2018 and December 2023. The study aimed to assess the outcomes of this subset of patients. The institutional review board (IRB) of Main Line Health Hospitals approved the study protocol (IRB 45CFR164.512), and informed consent was waived due to the retrospective nature of the study.
Clinical and procedural data were extracted from patient medical records. All participants underwent pre-procedural transthoracic and/or transesophageal echocardiography, as well as multi-detector computed tomography (MDCT) for TAVI assessment. The primary study endpoint was 30-day and follow-up mortality. Secondary outcomes included Valve Academic Research Consortium (VARC)-3 technical success, VARC-3 device success, and VARC-3 early safety.
Definitions
Clinical information followed standardized definitions of data elements of the STS/ACC TVT (Society of Thoracic Surgeons [STS]/American College of Cardiology [ACC] Transcatheter Valve Therapy) Registry.14 The estimated glomerular filtration rate (eGFR) was estimated with the 2021 CKD-EPI (Chronic Kidney Disease Epidemiology Collaboration) creatinine equation.15 SVD was defined according to modified VARC-3 criteria.16 Severe SVD was identified by an increase of at least 20 mm Hg in the mean transvalvular gradient compared with the echocardiographic assessment conducted 1 to 3 months after the procedure, resulting in a mean gradient of 30 mm Hg or more. Additionally, severe SVD included the new onset or a rise of at least 2 grades in intraprosthetic aortic regurgitation, leading to severe regurgitation.
Patient-prosthesis mismatch (PPM) was evaluated using the indexed effective orifice area (iEOA) of the transcatheter heart valve (THV), calculated by dividing the EOA by the patient’s body surface area.17 Based on VARC-3 guidelines, PPM is diagnosed in patients with a body mass index (BMI) greater than or equal to 30 kg/m² when the iEOA is less than or equal to 0.70 cm²/m², and severe PPM when the iEOA is less than or equal to 0.55 cm²/m². In patients with a BMI less than 30 kg/m², PPM is defined as an iEOA below 0.85 cm²/m², with severe PPM occurring when the iEOA is under 0.65 cm²/m².16
Statistical methods
Categorical data were expressed as frequencies and percentages. The normality of continuous variables was assessed using the Kolmogorov-Smirnov test. Normally distributed variables were reported as means and SDs, while non-normally distributed variables were presented as medians and IQR.
All statistical tests were 2-sided, and results were considered significant at a P-value of less than 0.05. Data were analyzed using R software version 4.4.1 (R Project for Statistical Computing) through RStudio. Upon request and subject to institutional approval, data supporting the conclusions of the study can be made available. The study followed the STROBE guidelines (STrengthening the Reporting of Observational Studies in Epidemiology) for observational research.18
Results
Between 2018 and 2023, our center performed a total of 1476 TAVI procedures. Of these, 15 patients underwent ViV-TAVI in a previously implanted stentless valve. The mean age of the patients was 78.1 ± 9.3 years, and 6 (40%) were female. The median STS-PROM score was 4.30 (3.05, 6.75). Three (20%) patients had a history of coronary artery bypass grafting (CABG), and 2 (13.3%) had undergone prior percutaneous coronary intervention (PCI). The mean time between SAVR and TAVI was 14.7 ± 3.9 years. Eight (53.3%) patients presented with severe aortic regurgitation and 5 (33.3%) had severe aortic stenosis, while 2 (13.3%) a mixed etiology. A summary of all baseline characteristics can be found in Table 1.
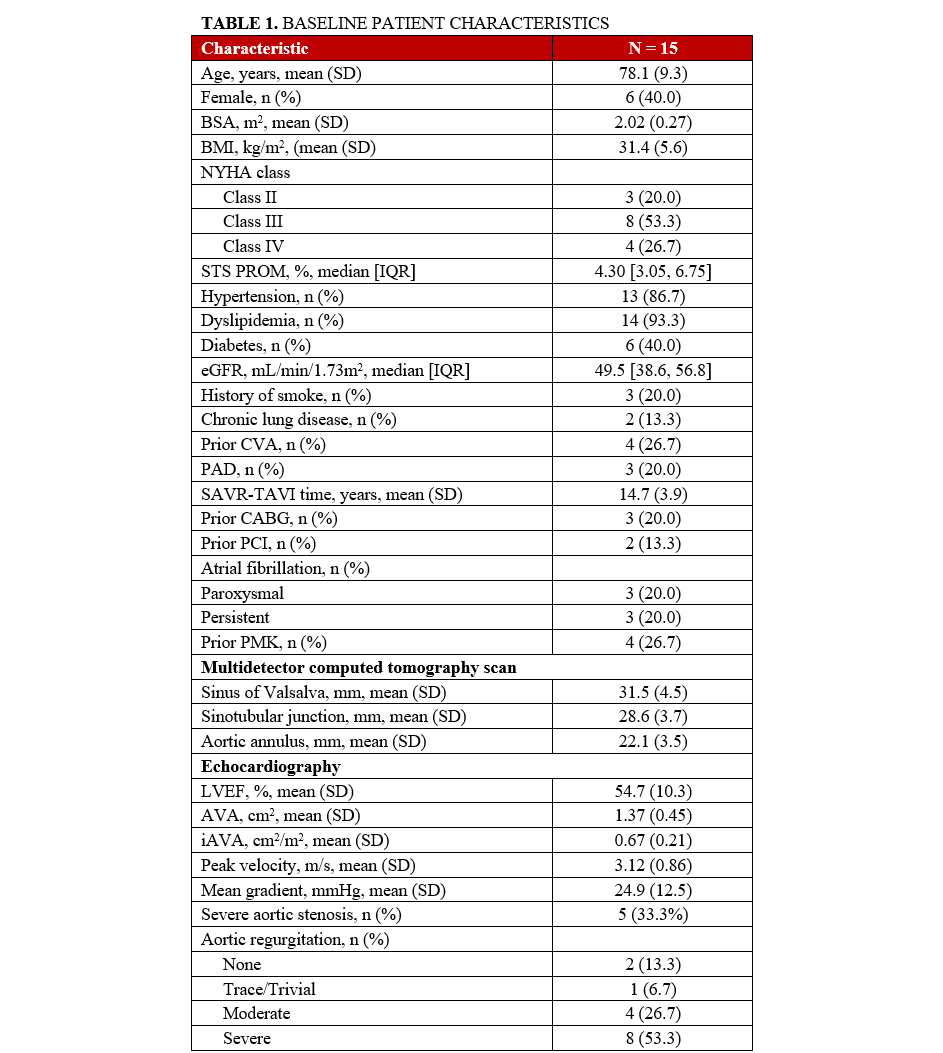

Most patients (n = 9, 60%) had previously received a Freestyle valve (Medtronic), followed by 3 patients (20%) with a Toronto Root (St. Jude Medical), 2 patients (13.3%) with a Prima/Prima Plus valve (Edwards Lifesciences), and 1 patient with a 3F Enable valve (ATS Medical).
A balloon-expandable valve was used in 9 (60%) patients, while a self-expandable valve was deployed in the remaining 6 (40%) patients. Three high-risk patients for coronary obstruction underwent concomitant PCI with the snorkel technique to protect the coronary ostia. There were no cases of surgical conversion. However, 1 patient experienced THV malposition, requiring the deployment of a second valve; the THV migrated to the ascending aorta after post-dilatation and was snared to the ascending aorta to avoid further migration. A second THV was then placed across the previous stentless valve. During the postoperative period, this patient experienced a stroke and required the implantation of a new permanent pacemaker for a third-degree atrioventricular block. She was discharged and remains alive as of February 2025.
Perioperative complications were rare and are detailed in Table 2. There was 1 case of postoperative stroke, 1 case of need for new pacemaker implantation, and 1 death at 30 days. VARC-3 technical success was achieved in 87.6% (n = 13) of patients, device success in 80% (n = 12), and early safety in 73.3% (n = 11).
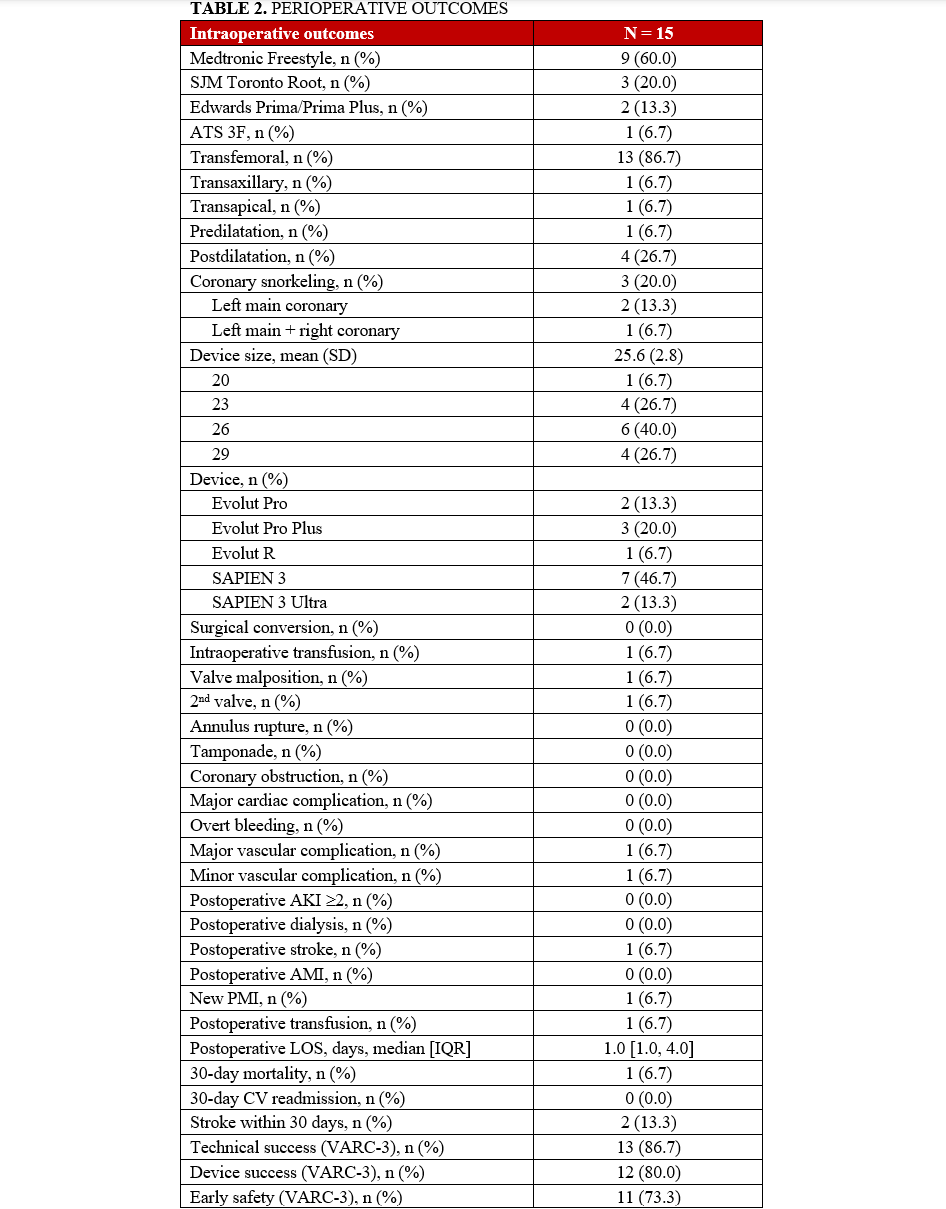

At discharge and at the 1-year follow-up, echocardiograms demonstrated satisfactory hemodynamic outcomes, with no instances of PVL greater than 2, as shown in Table 3. The median follow-up period was 2.9 years (range, 1.3-4.3 years), during which 4 patients died. All other follow-up complications are summarized in Table 4.
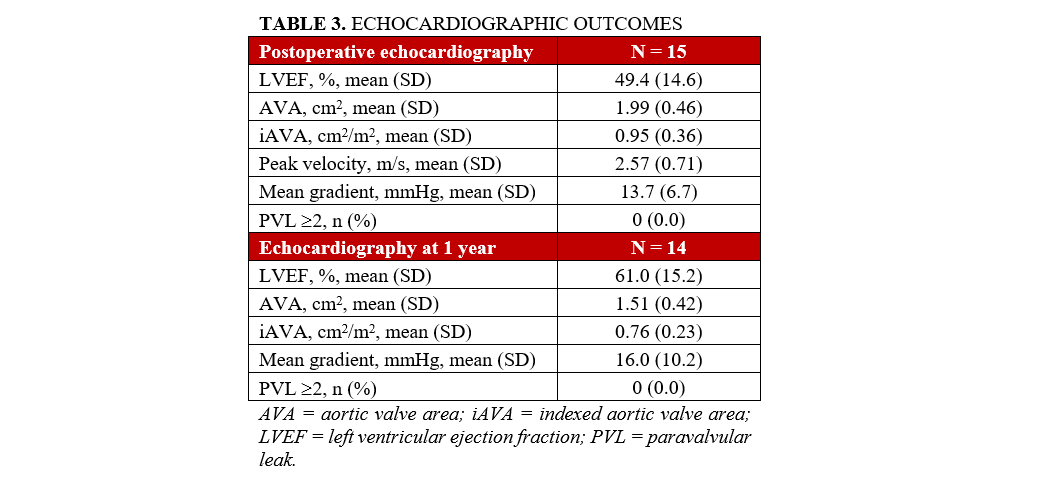
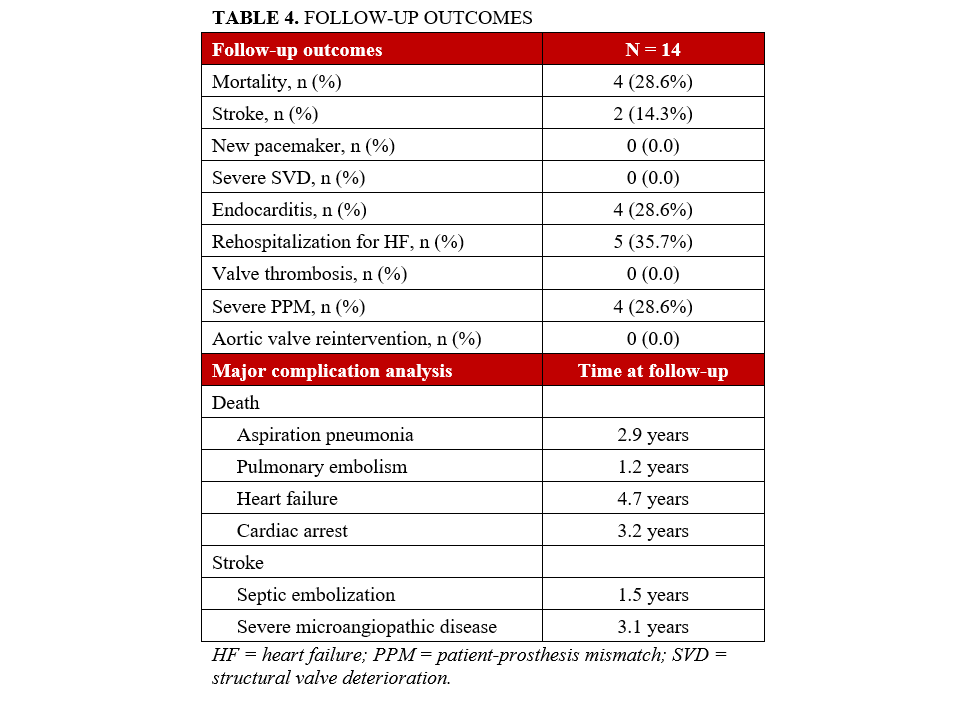
Discussion
We aimed to assess the outcomes of patients with stentless bioprosthesis failure undergoing ViV-TAVI using VARC-3 criteria. The key findings of this series can be summarized as follows: (1) coronary obstruction protection using the snorkel technique was employed in 3 patients with low-lying coronary ostia; (2) 1 case of THV dislocation occurred, requiring the deployment of a second THV; and (3) despite these challenges, satisfactory VARC-3 outcomes were achieved.
Failure and degeneration of surgically implanted stentless valves are unavoidable over time. In this series, an average of almost 15 years elapsed between SAVR and TAVI, in line with recent publications on this topic.4,13 Although current guidelines recommend redo surgical valve replacement, this approach carries significant risks, with morbidity and mortality rates often underrepresented by the surgical risk scores. For patients at higher surgical risk, ViV-TAVI offers an alternative in selected patients, potentially reducing the need for multiple surgeries over a lifetime. In fact, ViV-TAVI is increasingly utilized to treat SVD following SAVR.19 However, it presents unique technical challenges, such as a higher risk of coronary obstruction compared with TAVI for native aortic stenosis.20 This occurs because the leaflets of the surgical aortic valve extend beyond the aortic root and above the sinotubular junction. During ViV-TAVI, the valve leaflets are pushed outward, forming a cylinder that can trap the sinuses and block blood flow to the coronary arteries. This is further enhanced in narrow aortic roots and non-coaxial implant of the previous bioprosthesis. Duncan et al reported from the Valve-in-Valve International Data (VIVID) registry that coronary obstruction was 4 times more likely in failing stentless ViV-TAVI than in stented valves, and 8 times more common in non-homograft stentless cases than in failing homograft valves.9 Previous analyses from the VIVID registry also highlighted anatomical risk factors for coronary obstruction in ViV-TAVI, including stentless bioprostheses and stented bioprostheses with externally mounted leaflets.20
To evaluate the risk of coronary obstruction in ViV-TAVI, a multidetector computed tomography scan is recommended to measure the valve-to-coronary and valve-to-sinotubular junction distances. A measurement of less than 3 mm indicates a high risk for coronary obstruction.21 Several strategies have been developed to mitigate this risk, including the BASILICA (Bioprosthetic or native Aortic Scallop Intentional Laceration to prevent Iatrogenic Coronary Artery obstruction) technique, which involves intentionally lacerating the valve leaflets to prevent them from obstructing the coronary arteries during TAVI.22-24 Another strategy considers coronary protection with guidewires and stents, which involves placing guidewires and undeployed stents in the coronary arteries before TAVI to address any obstruction that might occur, or deploy the coronary stent as prevention with the chimney or snorkel techniques.25 The latter was deemed necessary in 3 patients and no cases of coronary obstruction were reported.
The use of stented bioprostheses facilitates THV deployment in ViV-TAVI, as most come with radiopaque markers that assist in precise placement. This benefit is diminished in stentless or homograft valves, where such markers are absent (Figure). Besides, there may be a lack of structural support for valve anchoring, particularly in cases of aortic regurgitation and no calcification.12 Data from the VIVD registry showed that stentless ViV-TAVI had a nearly doubled malposition rate and more than a double need for a second prosthesis compared with stented cases.9 Device oversizing may have played a role in malpositioning, especially given the lack of established sizing guidelines for failing stentless bioprostheses.
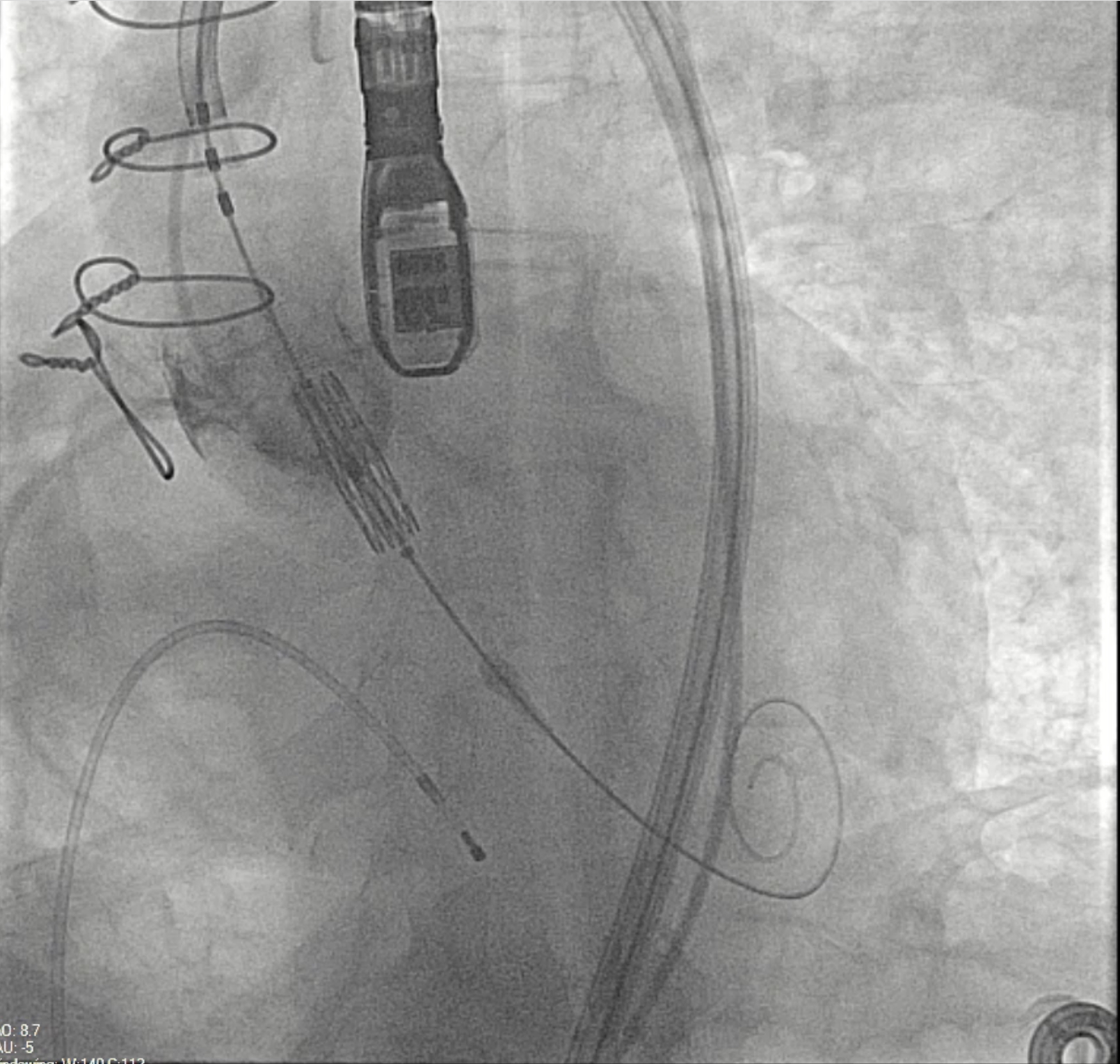
Despite the complexity of the procedure, there was only 1 instance of THV migration intraoperatively, while postoperative complications were minimal. These included 1 major and 1 minor vascular complication, 1 case of postoperative stroke, and 1 need for new pacemaker implantation. Increased clinical experience has demonstrated similarly favorable outcomes in line with recent studies.4,13 However, earlier publications reported higher perioperative complication rates and lower success according to VARC criteria.26,27 Huczek et al found a 15% rate of incorrect THV positioning and 5% procedural mortality, and reported a VARC-2 device success rate of 50% with 74% VARC-2 early safety and 72% VARC-2 clinical efficacy.27 Choi et al reported a 7.55% rate of THV procedural embolization.26
Finally, a direct comparison between the 2 THV was not feasible in this analysis. However, the low rates of postoperative complications suggest favorable outcomes with either valve type when the appropriate valve is selected for the right patient using an individual optimized strategy. Currently, there is no definitive evidence to guide THV selection for redo-TAVR. The choice depends on the index THV type, the failure mechanism, and anatomical relationships, including left ventricular outflow tract dimensions, native annulus, sinuses of Valsalva, sino-tubular junction, and ascending aorta. Coronary access impairment is a key consideration, particularly in cases with a narrow sinus of Valsalva or a short distance from the coronary ostia to the index THV frame, where balloon-expandable valves (BEV) may provide an advantage.19 On the other hand, self-expandable valves (SEV) may be beneficial for patient-prosthesis mismatch at index TAVR or for procedures with high risk for elevated residual gradients.19 The design of the original THV affects the neoskirt height, with supra-annular leaflet positioning in a tall stent frame increasing the obstruction risk.28,29 This height varies across THV sizes and generations. THV selection and implantation technique for redo-TAVR can mitigate risk, such as by implanting a short-frame THV at a lower position within a tall-frame THV. Conversely, using a tall-frame THV in a failed tall-frame THV may result in a higher neoskirt and greater obstruction risk.19
Of note, Moubarak et al previously compared SEV and BEV THVs in ViV-TAVI with stentless valves.30 The authors showed that ViV-TAVI for failed stentless bioprostheses using SEV had higher rates of periprocedural complications, including device malposition, need for post-deployment balloon dilation, and requirement of a second transcatheter device. However, both BEV and SEV demonstrated improved aortic valve function at 30-day and 1-year follow-up. Additionally, ViV-TAVI for degenerative stentless bioprostheses was linked to acceptable survival rates at 1 and 3 years, with no significant survival difference between BEV and SEV groups.
Limitations
The main limitation of this study arises from its retrospective design, which may not account for all potential confounding factors. Additionally, the single-center setting may limit the broader applicability of these findings to a wider population. This limitation also highlights the need for long-term follow-up, as it may reveal important differences in late survival outcomes. The study's small sample size and the selected cohort of patients further limit the generalizability of its findings, as the outcomes observed may not fully represent broader patient populations.
Conclusions
Careful patient selection is critical for ViV-TAVI procedures because of the high risks of complications, increased by the presence of a stentless valve in addition to the standard risks associated with the ViV procedure. This cohort of patients was highly comorbid and symptomatic but was associated with acceptable early VARC-3 outcomes; however, significant complications were reported at mid-term follow-up. Larger studies are warranted to confirm these findings.
Affiliations and Disclosures
Massimo Baudo, MD1; Serge Sicouri, MD1; Yoshiyuki Yamashita, MD, PhD1,2; Dimitrios Magouliotis, MD, PhD, MSc1; Francesco Cabrucci, MD1; Scott M. Goldman, MD2; Roberto Rodriguez, MD2; Eric M. Gnall, DO3; Paul M. Coady, MD3; William A. Gray, MD1,3; Sandro Gelsomino, MD, PhD4; Basel Ramlawi, MD1,2
Dr Gelsomino and Dr Ramlawi share equal senior authorship.
From the 1Department of Cardiac Surgery Research, Lankenau Institute for Medical Research, Main Line Health, Wynnewood, Pennsylvania; 2Department of Cardiac Surgery, Lankenau Heart Institute, Main Line Health, Wynnewood, Pennsylvania; 3Department of Interventional Cardiology, Lankenau Heart Institute, Main Line Health, Wynnewood, Pennsylvania; 4Cardiovascular Research Institute Maastricht - CARIM, Maastricht University Medical Centre, Maastricht, the Netherlands.
Disclosures: Dr Gray is a consultant for, and is on the advisory board of Philips, Medtronic, Boston Scientific, Surmodics, Encompass Vascular, eFemoral, Cagent, Sonovascular, Conformal Medical, Contego Medical, ReValve, Alucent, and Edwards LifeSciences; and holds stock with eFemoral, Encompass Vascular, Xenter, and Reflow Medical. Dr Ramlawi is a consultant for, and is on the advisory board of Medtronic Inc., Boston Scientific Corporation, AtriCure Inc., and Corcym Inc.
Address for correspondence: Massimo Baudo, MD, Department of Cardiac Surgery Research, Lankenau Institute for Medical Research, Main Line Health, 100 E Lancaster Avenue, Wynnewood, PA 19096, USA. Email: massimo.baudo@icloud.com; X: @Takotsubo91
References
1. Isaacs AJ, Shuhaiber J, Salemi A, Isom OW, Sedrakyan A. National trends in utilization and in-hospital outcomes of mechanical versus bioprosthetic aortic valve replacements. J Thorac Cardiovasc Surg. 2015;149(5):1262-1269.e3. doi:10.1016/j.jtcvs.2015.01.052
2. Tavakoli R, Danial P, Oudjana AH, et al. Biological aortic valve replacement: advantages and optimal indications of stentless compared to stented valve substitutes. A review. Gen Thorac Cardiovasc Surg. 2018;66(5):247-256. doi:10.1007/s11748-018-0884-3
3. Ali A, Halstead JC, Cafferty F, et al. Are stentless valves superior to modern stented valves? A prospective randomized trial. Circulation. 2006;114(1 Suppl):I535-540. doi:10.1161/CIRCULATIONAHA.105.000950
4. Lang FM, Mihatov N, Kriegel J, et al. Transcatheter aortic valve-in-valve implantation within stentless landing zones: Procedural insights from a single-center experience. Catheter Cardiovasc Interv. 2023;102(2):328-338. doi:10.1002/ccd.30755
5. Dvir D, Webb J, Brecker S, et al. Transcatheter aortic valve replacement for degenerative bioprosthetic surgical valves: results from the global valve-in-valve registry. Circulation. 2012;126(19):2335-2344. doi:10.1161/CIRCULATIONAHA.112.104505
6. Dvir D, Webb JG, Bleiziffer S, et al; Valve-in-Valve International Data Registry Investigators. Transcatheter aortic valve implantation in failed bioprosthetic surgical valves. JAMA. 2014;312(2):162-170. doi:10.1001/jama.2014.7246
7. Gulbins H, Reichenspurner H. Which patients benefit from stentless aortic valve replacement? Ann Thorac Surg. 2009;88(6):2061-2068. doi:10.1016/j.athoracsur.2009.06.060
8. Rodriguez-Gabella T, Voisine P, Puri R, Pibarot P, Rodés-Cabau J. Aortic bioprosthetic valve durability: incidence, mechanisms, predictors, and management of surgical and transcatheter valve degeneration. J Am Coll Cardiol. 2017;70(8):1013-1028. doi:10.1016/j.jacc.2017.07.715
9. Duncan A, Moat N, Simonato M, et al. Outcomes following transcatheter aortic valve replacement for degenerative stentless versus stented bioprostheses. JACC Cardiovasc Interv. 2019;12(13):1256-1263. doi:10.1016/j.jcin.2019.02.036
10. Miller M, Snyder M, Horne BD, et al. Transcatheter aortic valve-in-valve replacement for degenerated stentless bioprosthetic aortic valves: results of a multicenter retrospective analysis. JACC Cardiovasc Interv. 2019;12(13):1217-1226. doi:10.1016/j.jcin.2019.05.022
11. Cekmecelioglu D, Preventza O, Dougherty KG, et al. Transcatheter valve-in-valve implantation for degenerated stentless aortic bioroots. Ann Cardiothorac Surg. 2021;10(5):641-650. doi:10.21037/acs-2021-tviv-124
12. Baudo M, Cuko B, Ternacle J, et al. Transcatheter valve-in-valve interventions after aortic root replacement: a systematic review. Catheter Cardiovasc Interv. 2024;103(7):1101-1110. doi:10.1002/ccd.31027
13. Steul JH, Abdel-Wahab M, Stankowski T, et al. VARC-3 defined outcome of valve-in-valve transcatheter aortic valve implantation in stentless compared with stented aortic bioprostheses. Clin Res Cardiol. 2023. doi:10.1007/s00392-023-02347-5
14. Carroll JD, Mack MJ, Vemulapalli S, et al. STS-ACC TVT registry of transcatheter aortic valve replacement. J Am Coll Cardiol. 2020;76(21):2492-2516. doi:10.1016/j.jacc.2020.09.595
15. Inker LA, Eneanya ND, Coresh J, et al; Chronic Kidney Disease Epidemiology Collaboration. New creatinine- and cystatin c-based equations to estimate GFR without race. N Engl J Med. 2021;385(19):1737-1749. doi:10.1056/NEJMoa2102953
16. Généreux P, Piazza N, Alu MC, et al. Valve Academic Research Consortium 3: updated endpoint definitions for aortic valve clinical research. J Am Coll Cardiol. 2021;77(21):2717-2746. doi:10.1016/j.jacc.2021.02.038
17. Pibarot P, Dumesnil JG. Hemodynamic and clinical impact of prosthesis-patient mismatch in the aortic valve position and its prevention. J Am Coll Cardiol. 2000;36(4):1131-1141. doi:10.1016/s0735-1097(00)00859-7
18. Vandenbroucke JP, von Elm E, Altman DG, et al. Strengthening the Reporting of Observational Studies in Epidemiology (STROBE): explanation and elaboration. PLoS Med. 2007;4(10):e297. doi:10.1371/journal.pmed.0040297
19. Tarantini G, Sathananthan J, Fabris T, et al. Transcatheter aortic valve replacement in failed transcatheter bioprosthetic valves. JACC Cardiovasc Interv. 2022;15(18):1777-1793. doi:10.1016/j.jcin.2022.07.035
20. Ribeiro HB, Rodés-Cabau J, Blanke P, et al. Incidence, predictors, and clinical outcomes of coronary obstruction following transcatheter aortic valve replacement for degenerative bioprosthetic surgical valves: insights from the VIVID registry. Eur Heart J. 2018;39(8):687-695. doi:10.1093/eurheartj/ehx455
21. Tarantini G, Dvir D, Tang GHL. Transcatheter aortic valve implantation in degenerated surgical aortic valves. EuroIntervention. 2021;17(9):709-719. doi:10.4244/EIJ-D-21-00157
22. Khan JM, Dvir D, Greenbaum AB, et al. Transcatheter laceration of aortic leaflets to prevent coronary obstruction during transcatheter aortic valve replacement: concept to first-in-human. JACC Cardiovasc Interv. 2018;11(7):677-689. doi:10.1016/j.jcin.2018.01.247
23. Khan JM, Babaliaros VC, Greenbaum AB, et al. Preventing coronary obstruction during transcatheter aortic valve replacement: results from the multicenter international BASILICA registry. JACC Cardiovasc Interv. 2021;14(9):941-948. doi:10.1016/j.jcin.2021.02.035
24. Westermann D, Ludwig S, Kalbacher D, et al. Prevention of coronary obstruction in patients at risk undergoing transcatheter aortic valve implantation: the Hamburg BASILICA experience. Clin Res Cardiol. 2021;110(12):1900-1911. doi:10.1007/s00392-021-01881-4
25. Ibrahim H, Chaus A, Alkhalil A, Prescher L, Kleiman N. Coronary artery obstruction after transcatheter aortic valve implantation: past, present, and future. Circ Cardiovasc Interv. 2024;17(6):e012827. doi:10.1161/CIRCINTERVENTIONS.123.012827
26. Choi CH, Cao K, Malaver D, et al. Redo-aortic valve replacement in prior stentless prosthetic aortic valves: transcatheter versus surgical approach. Catheter Cardiovasc Interv. 2022;99(1):181-192. doi:10.1002/ccd.29921
27. Huczek Z, Grodecki K, Scisło P, et al. Transcatheter aortic valve-in-valve implantation in failed stentless bioprostheses. J Intervent Cardiol. 2018;31(6):861-869. doi:10.1111/joic.12540
28. Akodad M, Sellers S, Gulsin GS, et al. Leaflet and neoskirt height in transcatheter heart valves: implications for repeat procedures and coronary access. JACC Cardiovasc Interv. 2021;14(20):2298-2300. doi:10.1016/j.jcin.2021.07.034
29. Rogers T, Greenspun BC, Weissman G, et al. Feasibility of coronary access and aortic valve reintervention in low-risk TAVR patients. JACC Cardiovasc Interv. 2020;13(6):726-735. doi:10.1016/j.jcin.2020.01.202
30. Moubarak G, Salih M, Eisenga J, et al. Transcatheter valve-in-valve replacement with balloon- versus self-expanding valves in patients with degenerated stentless aortic bioprosthesis. Am J Cardiol. 2024;230:50-57. doi:10.1016/j.amjcard.2024.08.001