Parallel Cholesterol Crystals: A Sign of Impending Plaque Rupture?
ABSTRACT: Background. Currently, there are no available methods that can reliably predict when or if an atheroma will rupture. Recent reports suggest cholesterol crystals (CCs), present within the necrotic core, are sharp and can penetrate and disrupt the fibrous cap, contributing to plaque rupture. Our aim is to show CC, normally distributed at random within the necrotic core, often develop a parallel configuration at the site of plaque disruption (PD) which may be a sign of impending plaque rupture. Methods and Results. The coronary arteries of 83 patients who died of acute coronary disease (ACD) were injected with a colored barium gelatin mass. The arteries were dissected, decalcified, cut at 2–3 mm intervals, and all segments mounted for microscopic study. All segments were reviewed to identify PDs and to determine the frequency of parallel cholesterol crystals (P-CCs) at the site of these PDs. There were 215 separate PDs in 83 patients, with 64 (77%) patients showing more than one PD. P-CCs were present in 126 (59%) of all PDs regardless of plaque size or the severity of luminal stenosis and were present with or without luminal thrombosis. Luminal thrombosis was present in 105 PDs (49%), primarily in those segments showing significant luminal stenosis. There was no significant difference in the frequency of luminal thrombosis in those PDs with or without P-CCs. Conclusions. P-CCs are commonly present at the site of PD and may contribute to PD by penetrating and disrupting the fibrous cap. The ability to recognize this parallel configuration may help to identify plaques with impending plaque rupture.
J INVASIVE CARDIOL 2010;22:406–411
Key words: pathology, plaque, thrombosis, cholesterol crystals, vulnerable plaque
Plaque rupture is currently unpredictable and is the most frequent cause of acute coronary events,1,2 but the precise mechanism by which plaque rupture occurs remains to be determined.3 The vulnerable plaque, the thin cap fibroatheroma (TCFA), can now be identified by various scanning techniques, but this identification is not a reliable indicator of impending rupture.3 Many TCFAs are found incidentally at autopsy, showing that these pathological features do not predict when or if such plaques will rupture.2,4 Further, the core size and fibrous cap thickness are not statistically related, and many plaques rupture or erode without significant underlying luminal stenosis.3,5,6 Small atheromas with thin caps are just as likely to erode and rupture as large atheromas. At present, there are no reliable objective measures or indicators of impending plaque rupture that can be used by clinicians in the management of patients with coronary disease.1–3 Recent studies by Abela et al have focused on the possible pathogenetic potential of CCs, a common component of the atheromatous core, as a possible contributor to plaque rupture.7–9 Abela’s in vitro experiments, which used a test tube with the open end covered by a biologic membrane, showed that CCs form and expand rapidly in supersaturated solutions of free cholesterol, expanding the volume by as much as 45%.7 The conversion of free cholesterol to crystals is similar to the expansion that occurs when water changes to ice.7 The CCs formed in this manner have sharp, needle-like tips, come together to form a parallel configuration and expand to puncture the overlying membrane.7,8 Abela’s experiments demonstrated the pathogenetic potential of CCs to contribute to disruption of the fibrous cap. Subsequent study of carotid endarterectomy specimens, plus Abela’s postmortem studies of the coronary arteries, confirmed that CCs are frequently present at the site of PDs and may play a role in causing these PDs.9 Until Abela’s work, the in vivo physical characteristics of CCs within an atheroma, specifically whether these crystals contributed to or were capable of disrupting the fibrous cap, had not yet been determined. We have previously noted the presence of CCs, particularly a peculiar parallel configuration of CCs at the site of many PDs, but until Abela’s work, we did not know whether these crystals were actually as sharp as they appear and whether they could disrupt tissue and contribute to PD.10 Our aim in this study is to extend and support Abela’s observations by reporting our findings in a large number of PDs in patients who died of ACD, to determine the frequency of P-CCs at the site of PD and to find out whether this peculiar parallel configuration could be a reliable sign of impending plaque rupture.
Methods
Study population. The coronary arteries of 83 patients who died of ACD and 22 control patients who died of non-coronary causes were examined to determine the presence of P-CCs at the site of all PDs. The clinical features of these patients and the postmortem technique of study have been reported previously.6,11 The acute coronary syndromes included were cardiogenic shock, sudden cardiac death (SCD) with or without acute myocardial infarction and cardiac rupture associated with acute infarction.
Injection technique. All hearts were obtained fresh and uncut at the autopsy table, and the coronary arteries were injected with a colored barium gelatin mass. The coloring material withstands histologic processing and facilitates the accurate identification of PDs and P-CCs.6 The hearts were then fixed in formalin, the coronary arteries dissected, decalcified, cut at 2–3 mm intervals and all sections mounted for microscopic study. A total of 7,143 segments, an average of 86 per heart, were examined histologically, including 4–6 subserial sections taken from each segment.
Plaque disruption and thrombosis. A plaque disruption was defined as any breach of endothelial integrity including plaque rupture, plaque fissures and plaque erosion.12 Plaque rupture was defined as colored injection mass penetrating deep into the necrotic core, whereas plaque erosion was defined as injection mass being localized to the subendothelial region without penetration into the necrotic core. Any PDs that extended into contiguous segments were counted as one PD. The term thrombosis refers to an intraluminal thrombus, whether totally occlusive or nonocclusive, and does not include intra-intimal thrombi. Thromboses without evidence of a plaque rupture or the presence of colored injected mass within the necrotic core were considered to have developed on an erosion. CCs dissolve on histologic processing but leave an outline or cleft on histologic sections, showing their location at the time of death.
Statistical method. Statistical analysis of data presented in this report utilized chi-square and Fisher’s exact probability tests; p-values
Results
Demographics. There were 64 men, 30–87 years, mean age 56 years, and 19 women 49–94 years, mean age 70 years, included in the study. One patient who had bypass surgery is included for illustration purposes. Twenty-two patients, 3 women 15–67 years of age, mean age 47 years, and 19 men 19–74 years of age, mean age 43 years, served as controls. Five control patients died as a result of accidents or trauma, 5 of noncoronary cardiomyopathy, 3 of cancer, 2 of suicide, 2 of stroke and 5 of miscellaneous causes.
Frequency of P-CCs. Table 1 compares the overall frequency of P-CCs and luminal thrombosis at the site of 215 PDs found in 83 patients. Multiple PDs were present, with 64 (77%) patients having more than one PD. Plaque rupture accounted for 70% of PDs, and 30% were plaque erosions, consistent with previous reports.3,13 There were many PDs that were not considered to be the culprit lesion, were not associated with either thrombosis or significant stenosis and were probably clinically silent lesions.6,13,14 P-CCs were associated with 126 (59%) of all PDs, and of these, 100 (79%) were plaque ruptures. Luminal thrombosis was present in 105 (49%) of all PDs, and of these, 66 (63%) were associated with plaque rupture. These results show plaque rupture is the most common type of PD, and the majority of plaque ruptures are associated with P-CCs at the rupture site.
Table 1. Comparison of the frequency of P-CCs, plaque ruptures, erosions and luminal thrombosis in 83 patients who died of acute coronary disease
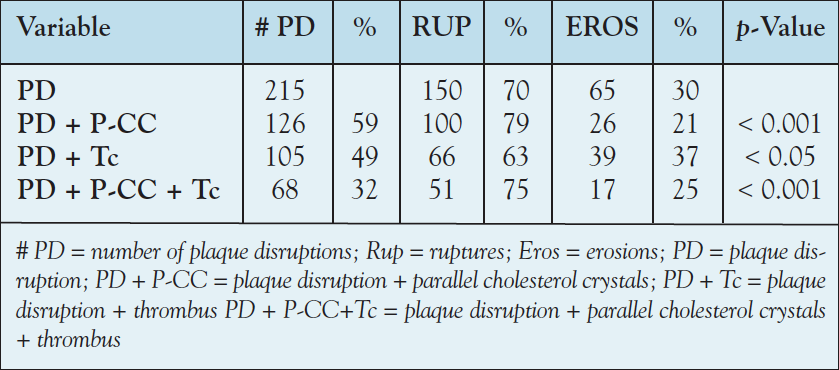
P-CCs and thrombosis. Similarly, 68 (65%) of the 105 PDs with luminal thrombosis were associated with P-CC, with the majority, 75%, associated with plaque rupture. However, there was no significant difference in the frequency of luminal thrombosis in those PDs, with or without P-CCs, suggesting no direct relationship between PDs with P-CCs and luminal thrombosis. These results are in contrast to those of Abela who found a strong relationship between plaque rupture, CCs and thrombosis.9 This difference is likely related to the high frequency of PDs in the present study, particularly PDs in areas of insignificant stenosis found by extensive histologic examination of the entire coronary tree. Plaque rupture and plaque erosions. Although erosions were infrequent compared to plaque rupture, 60% (39/65) of all erosions were associated with luminal thrombosis compared to 44% (66/150) of plaque ruptures; p ≤ 0.05. This finding may be explained by the fact that many plaque erosions were associated with significant luminal stenosis, whereas there were many plaque ruptures without thrombosis in segments without significant stenosis. Plaque erosions were found in 52 (32%) of 164 PDs in men compared to 13 (25%) of 51 in women, showing no significant gender difference in the frequency of plaque erosion. These results are different than those of Farb and coworkers,12 who noted younger women with ACD have more erosions than plaque ruptures, but our series did not include premenopausal women. Three control patients showed erosions without significant luminal stenosis or thrombosis and no P-CCs. One control patient had a ruptured plaque without P-CCs or thrombosis. P-CCs were found in very close proximity to the fibrous cap at the site of rupture or erosion. These results suggest that P-CCs may play an important role in the pathogenesis of some PDs, especially plaque rupture. However, a significant number of PDs showed neither P-CCs nor thrombosis, and other factors may play an important role in PD.
Illustrations. Figure 1 is an example of an apparent incipient fissure located in an area of insignificant luminal stenosis. This fissure may have been previously open but has resealed.15,16 This particular parallel configuration of the CC suggests the presence of increased intraplaque pressure pushing plaque contents toward the plaque shoulder.
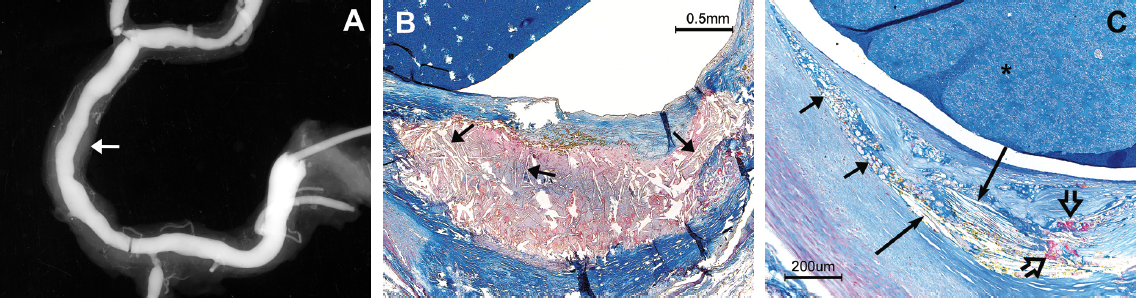
Figure 2 shows P-CCs within the fissure of a relatively small atheroma, demonstrating that PD is not always associated with luminal thrombosis.
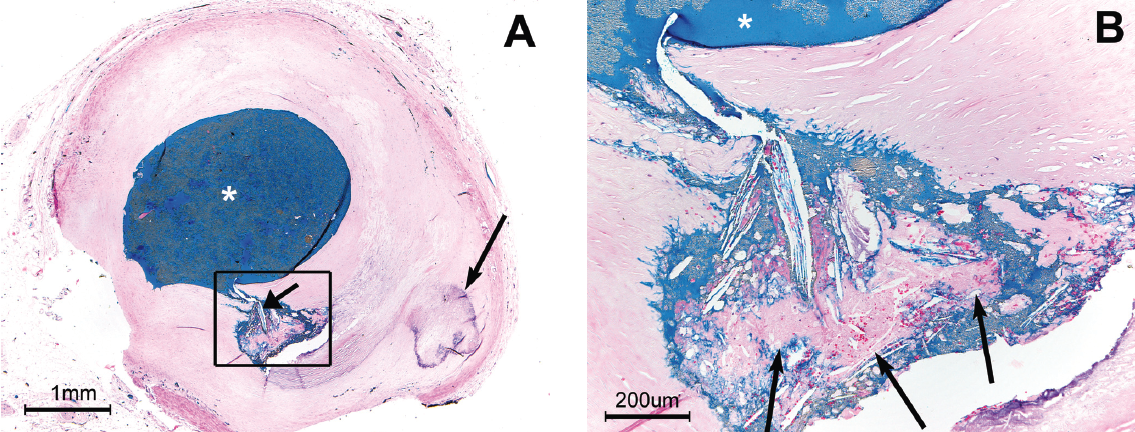
Figure 3 shows a plaque rupture in the proximal right coronary artery from a man who died of sudden cardiac death (SCD) while mowing the lawn. P-CC are present immediately upstream of this rupture, as well as at the site of the rupture. The rupture appears to be driven by high intraplaque pressure, producing an “explosive-like” image.
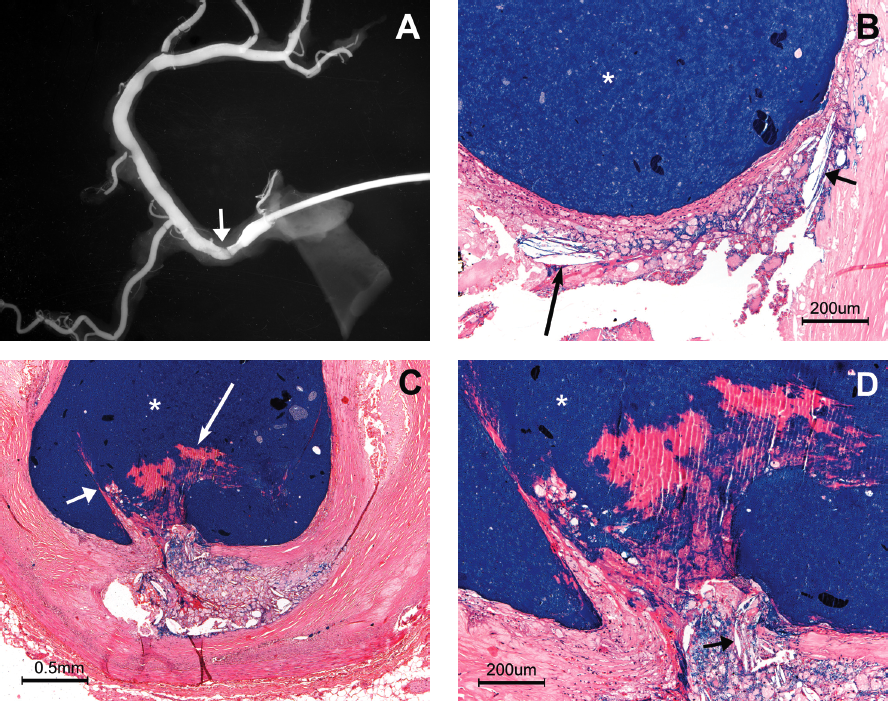
Figure 4 illustrates the relationship between P-CCs and luminal thrombosis in 4 different patients. In Figure 4A, the occlusive luminal thrombus is associated with endothelial erosion at the site of significant stenosis and associated with circumferential P-CCs at the endothelial surface. P-CCs are present within the body of the thrombus in Figure 4B, showing that the parallel configuration was present at the moment of thrombus formation. Figure 4C shows P-CCs immediately beneath the fibrous cap of a ruptured TCFA that is associated with luminal thrombosis. Figure 4D shows the relationship between plaque rupture and P-CCs mixed with thrombus at a point of high-grade stenosis, resulting in an obstructing thrombus.
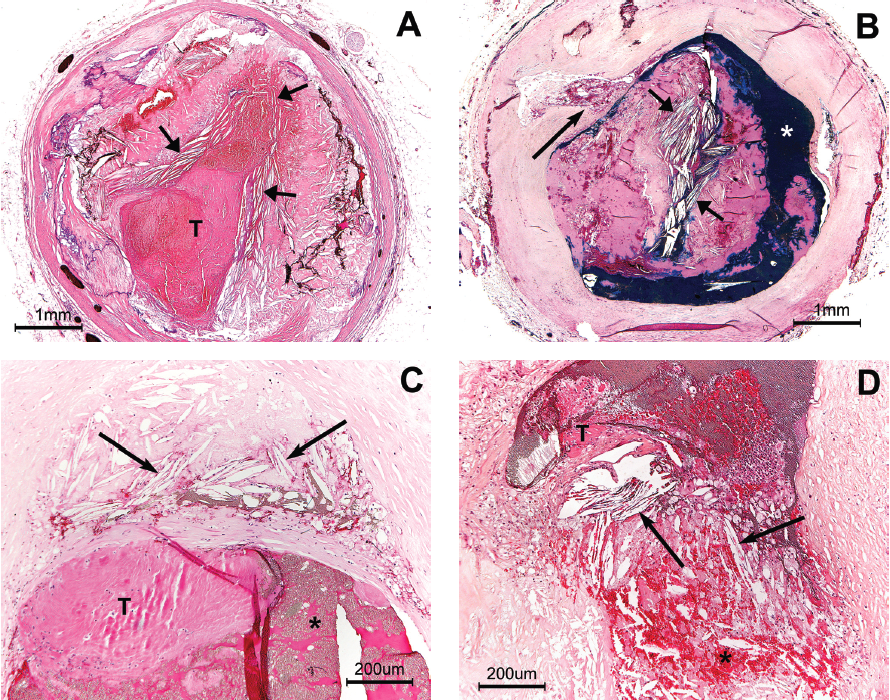
Discussion
Increased intraplaque pressure. Figures 1 and 3 both suggest atheromatous plaques develop an increase in intraplaque pressure. Previous investigators have postulated that plaque rupture may be caused by an increase in intraplaque pressure after observing the edges of the disrupted fibrous cap pointing outward into the lumen rather than inward to the necrotic core, as would be expected if the plaque rupture were due to external stress on the fibrous cap.17–19 Constantinides observed plaque rupture to be an almost explosive force, tearing apart the overlying fibrous cap.17 These histologic features suggest atheromas develop increased intraplaque pressure prior to plaque rupture. What factors may contribute to an increase in intraplaque pressure? An active and growing atheroma, enclosed by fibrous tissue, may be expected to result in an increase in intraplaque pressure merely on the basis of expanding volume. Abela’s in vitro experiments noted the formation of CCs is associated with a significant increase in volume, and the rate and volume of expansion correlated directly with the amount of free cholesterol.7,9 This volume expansion, created by the formation of the CCs, can be expected to increase intraplaque pressure and place increased internal stress on all surrounding tissue, including the overlying fibrous cap. Abela also showed that CCs can form rapidly and result in a sudden increase in intraplaque pressure.7,8 A rapid increase in intraplaque pressure could contribute to sudden plaque rupture and the onset of acute coronary events without warning.7 Guyton noted that lesions with CCs have high levels of free cholesterol and that large plaques are not a requirement for CC formation.20 Active, expanding, progressive atherosclerosis and the associated formation of CCs are two factors that may contribute to an increase in intraplaque pressure. When CCs develop a parallel configuration, it suggests there is an increase in intraplaque pressure, similar to Abela’s in vitro observations.7,8 We found P-CCs at the rupture site of both large and small atheromas, suggesting that all atheromas may develop increased intraplaque pressure. CCs are usually dispersed throughout the atheromatous core in a random fashion, with the crystals pointing in all directions (Figure 1) in plaques without disruption. P-CCs may be a sign of increased intraplaque pressure and may act much like a battering ram in contributing to PD. If this reasoning is correct, P-CCs may prove to be a reliable sign of an actively growing, expanding plaque and a sign of impending PD.
Hemodynamic/mechanical stress. Prior reports using computer simulation have suggested there is increased mechanical stess on the atheromatous plaque and its fibrous cap, particularly the plaque shoulder.20,21 If mechanical or external stress were a major factor in PD, we would not expect to find P-CCs at the site of plaque rupture nor embedded in the body of the thrombus, but rather distributed randomly. Further, we would not expect mechanical stress to play a role in those PDs involving small atheromas causing no significant stenosis or distortion of the artery wall. Mechanical/hemodynamic stress does not explain why CCs within the necrotic core assume a parallel configuration at the site of PD. Clinical significance. Determining when or if an atheroma, particularly a TCFA, will rupture has proven to be a daunting task.2,3 Improved scanning techniques, including Raman spectroscopy,22 Optical coherence tomography (OCT),23 elastography24 and other techniques allow the identification of the TCFA, but predicting impending rupture of the TCFA is not yet possible. CCs have been identified within the necrotic core in vivo using OCT, a promising technique that may prove to be useful in identifying P-CCs within the necrotic core.23 Further research is necessary to establish the significance of P-CCs and their role in PD. However, combining the findings of a large TCFA with P-CCs, located near or adjacent to the fibrous cap, may serve to identify a highly suspect vulnerable plaque. If subsequent research could establish P-CCs as a sign of impending PD, this could assist the clinician in the management of high-risk patients. For example, statin drugs have been shown to inhibit CC formation, and this reduction may be a factor in the reduction of heart attacks in patients receiving statins.25 Identifying P-CCs in close proximity to a TCFA could serve to focus attention on either the prevention or management of PD and justify intervention in asymptomatic, high-risk patients.26
Study limitations. This report was limited to the study of only those coronary segments showing PD, and did not assess the frequency of P-CCs in association with a TCFA that had not ruptured or eroded. The study population did not include premenopausal women, who are reported to have a high frequency of endothelial erosion, and the role of P-CCs in young women could not be assessed.
Summary and Future Directions
P-CCs are a consistent finding at the site of PD. It is not unreasonable to believe that improved scanning techniques will be able to recognize this configuration. In vitro and pathologic studies suggest that these crystals may act in a manner similar to a battering ram in contributing to PD. If P-CCs can be recognized, the clinician may be able to plan strategies to prevent acute coronary events. P-CCs may prove to be a reliable indicator of impending PD.
__________________________________________________
From the Heart Research Foundation of Sacramento, Sacramento, California. The author reports no financial relationships or conflicts of interest regarding the content herein. Manuscript submitted April 15, 2010, provisional acceptance given May 25, 2010, final version accepted June 2, 2010. Address for correspondence: Richard J. Frink, MD, FACP, FACC, Heart Research Foundation of Sacramento, 1007 39th Street, Sacramento, CA 95816-5502. E-mail: rjfrink@hrfsac.org
- Kullo IJ, Edwards WD, Schwartz RS. Vulnerable plaque: Pathobiology and clinical implications. Ann Intern Med 1998;129:1050–1060.
- Casscells W, Naghavi M, Willerson JT. Vulnerable atherosclerotic plaque: A multifocal disease. Circulation 2003;107:2072–2075.
- Naghavi M, Libby P, Falk E, et al. From vulnerable plaque to vulnerable patient: A call for new definitions and risk assessment strategies: Part I. Circulation 2003;108:1664–1672.
- Lee RT, Libby P. The unstable atheroma. Arterioscler Thromb Vasc Biol 1997;17:1859–1867.
- Mann JM, Davies MJ. Vulnerable plaque: Relation of characteristics to degree of stenosis in human coronary arteries. Circulation 1996;94:928–931.
- Frink RJ. Chronic ulcerated plaques: New insights into the pathogenesis of acute coronary disease. J Invasive Cardiol 1994;6:173–185.
- Abela GS, Aziz K. Cholesterol crystals cause mechanical damage to biological membranes: A proposed mechanism of plaque rupture and erosion leading to arterial thrombosis. Clin Cardiol 2005;28:413–420.
- Abela GS, Aziz K. Cholesterol crystals rupture biological membranes and human plaques during acute cardiovascular events — A novel insight into plaque rupture by scanning electron microscopy. Scanning 2006;28:1–10.
- Abela GS, Aziz K, Vedre A, et al. Effect of cholesterol crystals on plaques and intima in arteries of patients with acute coronary and cerebrovascular syndromes. Am J Cardiol 2009;103:959–968.
- Frink, RJ. Inflammatory atherosclerosis: Characteristics of the injurious agent. 1st ed. United States: Heart Research Foundation of Sacramento; 2002, p.16.
- Frink, RJ. Gender gap, inflammation and acute coronary disease: Are women resistant to atheroma growth? Observations at autopsy. J Invasive Cardiol 2009;21:270–277.
- Farb A, Burke AP, Tang AL, et al. Coronary plaque erosion without rupture into a lipid core: A frequent cause of coronary thrombosis in sudden coronary death. Circulation 1996;93:1354–1363.
- Rioufol G, Finet G, Ginon I, et al. Multiple atherosclerotic plaque rupture in acute coronary syndrome: A three-vessel intravascular ultrasound study. Circulation 2002;106:804–808.
- Fujii K, Kobayashi Y, Mintz GS, et al. Intravascular ultrasound assessment of ulcerated ruptured plaques: A comparison of culprit and nonculprit lesions of patients with acute coronary syndromes and lesions in patients without acute coronary syndromes. Circulation 2003;108:2473–2478.
- Mann J, Davies MJ. Mechanisms of progression in native coronary artery disease: Role of healed plaque disruption. Heart 1999;82:265–268.
- Burke AP, Kolodgie FD, Farb A, et al. Healed plaque ruptures and sudden coronary death: Evidence that subclinical rupture has a role in plaque progression. Circulation 2001;103:934–940.
- Constantinides P. Plaque fissures in human coronary thrombosis. J Atheroscler Res 1966;6:1–17.
- Friedman M. The coronary thrombus: Its origin and fate. Hum Pathol 1971;2:81–128.
- Horie T, Sekiguchi M, Hirosawa K. Relationship between myocardial infarction and preinfarction angina. Am Heart J 1978;95:81–88.
- Guyton JR, Klemp KF. Development of the lipid-rich core in human atherosclerosis. Arterioscler Thromb Vasc Biol 1996;16:4–11.
- Loree HM, Kamm RD, Stringfellow RG, Lee RT. Effects of fibrous cap thickness on peak circumferential stress in model atherosclerotic vessels. Circulation Research 1992;71:850–858.
- Römer TJ, Brennan JF III, Fitzmaurice M, et al. Histopathology of human coronary atherosclerosis by quantifying its chemical composition with Raman spectroscopy. Circulation 1998;97:878–885.
- Tearney G, Jang I-K, Bouma BE. Optical coherence tomography for imaging the vulnerable plaque. J Biomed Opt 2006;11:021002.
- Schaar JA, de Korte CL, Mastik F, et al. Characterizing vulnerable plaque features with intravascular elastography. Circulation 2003;108:2636–2641.
- Mason RP, Walter MF, Jacob RF. Effects of HMG-CoA reductase inhibitors on endothelial function: Role of microdomains and oxidative stress. Circulation 2004;109:34–41.
- Madjid M, Zarrabi A, Litovsky S, et al. Finding vulnerable atherosclerotic plaques: Is it worth the effort? Arterioscler Thromb Vasc Biol 2004;24:1775–1782.