ADVERTISEMENT
Barrier Functions of the Skin
Questions
1. What is the physical barrier of the skin?
2. How does the loss of the skin barrier affect water homeostasis?
3. How does the loss of the skin barrier compromise host immunity?
4. What is the role of the skin barrier in the regulation of body temperature?
Case Description
A 46-year-old male was admitted with a 90% total body surface area deep burn having been trapped in an industrial steam autoclave (Figure 1). Major complications included hemodynamic instability, sepsis, hypothermia, and skin fragility due to the loss of the barrier functions of the skin.
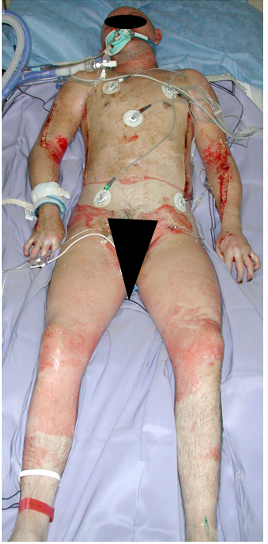
Q1. What is the physical barrier of the skin?
The skin forms a mechanical barrier against diverse forms of trauma. Where other land-living animals such as arthropods have adapted by possessing a rigid exoskeleton for protection, mammals have evolved a unique tough, flexible stratum corneum. The impact resistance of the stratum corneum is demonstrated by the failure of 2 mm-sized gold particles to penetrate the epidermis when fired from a gene gun at 500 psi.1
The combination of collagen and elastic fibers of the reticular dermis is responsible for the high tensile strength and flexibility necessary to absorb substantial mechanical forces delivered to the skin. This is facilitated by the thixotropic property of the ground substance of the extracellular matrix, which can reduce its viscosity in response to an applied mechanical force, thereby reducing friction between structures.2
Q2. How does the loss of the skin barrier affect water homeostasis?
Water homeostasis is primarily the function of the stratum corneum that impedes transepidermal water loss by integrating lipids with structural proteins to create an impermeable barrier. Large surface area burns can cause excessive water loss, dehydration, renal failure, and hemodynamic shock.
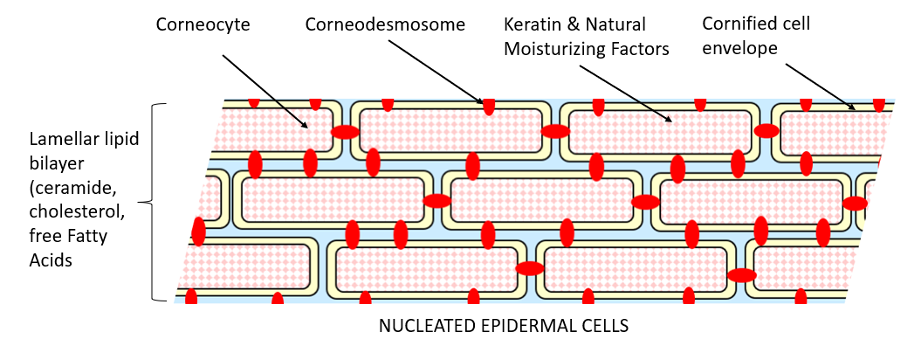
Eighty percent of the corneocytes are composed of keratin, whose filaments are aligned into disulfide cross-linked macrofibres.3 Keratohyalin granules of the stratum granulosum contain profilaggrin (which is cleaved to filaggrin required for aggregation of keratin filaments) and loricrin and involucrin (which cross-link under the mediation of calcium-dependent epidermal transglutaminase to form the tough thickened, cornified envelope of the corneocytes). Further breakdown of filaggrin leads to a mixture of amino acids collectively termed natural moisturizing factors that allows the cells to hold sufficient moisture to plasticize the keratin. The membrane-bound lamellar bodies in this granular layer secrete their lipid contents into the intercellular space between the stacked corneocytes and undergo further catabolic modification to give rise to an equimolar mixture of cholesterol, ceramides, and fatty acids. This mortar lipid allows a limited quantity of water in the vapor phase to pass through, resulting in insensible perspiration, permitting evaporative cooling while preventing major loss of water (Figure 2). Caspase activity induces apoptosis of keratinocytes. Finally, desmosomes are modified to corneodesmosomes, which undergo proteolysis at the skin surface allowing the corneocytes to be shed.
Important mediators of transcellular water flow and skin hydration are the aquaporins, a family of transmembrane proteins that facilitates transport of water and solutes, such as glycerol and urea, across cell membranes in response to changing osmotic gradients. It has been hypothesized that aquaporin-3 (AQP-3) could be stimulated by burn-related dehydration and play a vital role in retaining water. AQP-3 is absent in full-thickness burns.5
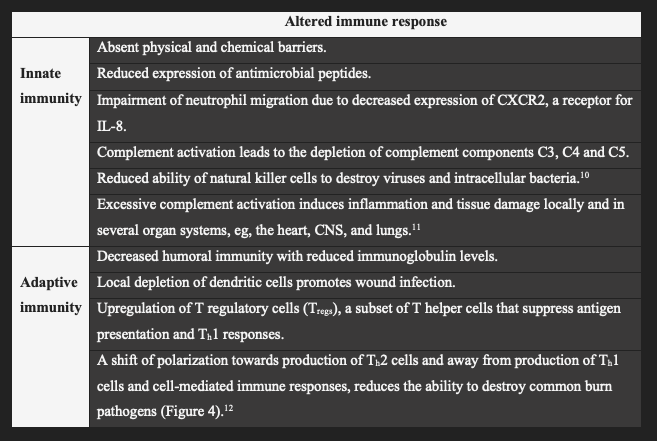
Q3. How does the loss of the skin barrier compromise host immunity?
Injury to the skin barrier can disrupt the innate and adaptive immune mechanisms (Table 1). The burn eschar is a nidus and portal for bacterial invasion, which is favored by removal of the mechanical barrier, the skin microbiome,6 and bactericidal lipids in the stratum corneum. Keratinocytes of the epidermis synthesize antimicrobial peptides, such as defensins that are packaged in the lamellar bodies of the stratum granulosum, forming a link between the antimicrobial and permeability barriers (Figure 3).
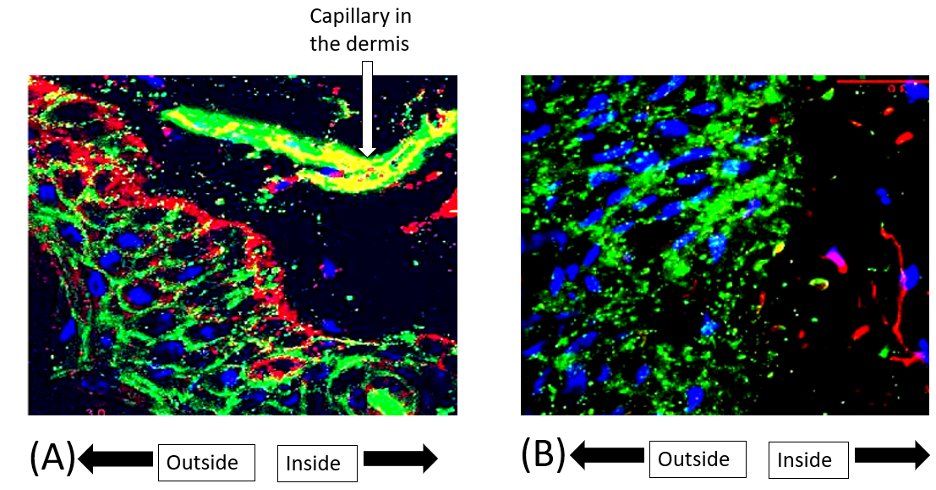
Beneath the stratum corneum, macrophages, neutrophils, and dendritic cells possess pattern recognition receptors, such as Toll-like receptors (TLRs), that bind pathogen-associated molecular patterns (PAMPS; eg, bacterial lipopolysaccharide) and damage-associated molecular patterns (DAMPS; eg, mitochondrial DNA and viral dsRNA). Ligand/receptor interaction (eg, TLR4 and lipopolysaccharide) causes nuclear translocation of the transcription factor NFkB and release of proinflammatory cytokines, resulting in inflammation that can progress to systemic inflammatory response syndrome, sepsis, and multiple organ failure.8 Production of IL-8 plays a pivotal role in the recruitment of neutrophils, which destroy microorganisms within a phagolysosome using lysosomal enzymes, and generate reactive oxygen species in the oxidative burst. Virally infected cells are targeted by natural killer cells, which release perforin that creates pores in the lipid bilayer through which proteases, called granzymes, enter to induce cell lysis or apoptosis. Another first line of defense is the complement system of more than 30 serum and cellular proteins whose principal role is to kill and opsonize microorganisms.
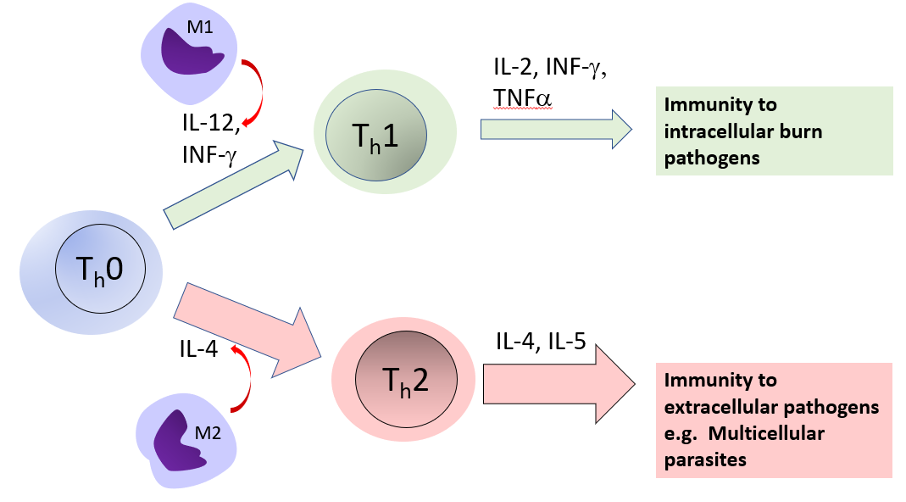
Adaptive immune responses are regulated by Langerhans cells, which capture, process, and present pathogens on MHC molecules to naïve T cells where they are primed to become effector (CD4 or helper Th) or CD8 (cytotoxic) T cells (Figure 4). The T-helper cells also activate naïve B cells in the lymph nodes to become effector B cells (plasma cells) that produce antibodies. Lymphocytes then migrate to the epidermis where they reside as memory B and T cells that can be rapidly reactivated.
Q4. What is the role of the skin barrier in the regulation of body temperature?
With large burns, heat preservation may be lost, particularly if the skin is excised to the level of the fascia. A temperature gradient of 2 to 4ºC normally exists between the skin and core tissues. This is regulated by the amount of blood flowing through the skin, which has an extensive superficial network of arterioles, venules, and capillaries and a deep network of veins with many arteriovenous anastomoses. When the core temperature rises, sympathetic activity to the skin decreases, resulting in vasodilation, facilitating convective heat transfer to the environment. Conversely, when the core temperature falls, increased sympathetic tone causes vasoconstriction, shunting blood away from the surface of the skin.13 Heat loss is assisted through evaporative cooling by the eccrine sweat glands as well as by evaporation, whereby patients can lose as much as 4000 mL/m2 of fluids per day.
Though patients compensate partly through an increased metabolic rate,14 hypothermia, defined as a core temperature less than 36°C, readily occurs in patients with large burns and poses a significant intraoperative risk. Shivering, which increases oxygen consumption, is a particular problem in patients predisposed to myocardial ischemia. Prolongation of coagulation times and platelet dysfunction can increase blood loss, and the combination of hypothermia, acidaemia, and coagulopathy is a lethal combination. Core temperature must be monitored throughout surgery and during transport. Maintenance of high ambient air temperature in the operating room, warming fluids, and the use of radiant heaters are effective in maintaining core temperature. A child’s head is about 20% of his/her body and can lose a proportional amount of heat if uncovered. When large wounds are finally resurfaced with a combination of skin grafts and scar, a lack of sweat glands may render the patient anhidrotic, causing distressing heat intolerance.15
Acknowledgments
Affiliations: Professor of Plastic and Reconstructive Surgery, Johns Hopkins University School of Medicine, Retired, Baltimore MD; Director, Johns Hopkins Burn Center, Retired, Baltimore, MD
Correspondence: Stephen M Milner, MBBS, BDS, DSc (Hon) FRCSE, FACS; stephenmilner123@gmail.com
Disclosure: The authors disclose no financial or other conflicts of interest.
References
1. Menon GK, Brandsma JL, Schwartz PM. Particle-mediated gene delivery and human skin: ultrastructural observations on stratum corneum barrier structures. Skin Pharmacol Physiol. 2007;20(3):141-147. doi:10.1159/000098165
2. Smit HJ, Strong P. Structural Elements of the Biomechanical System of Soft Tissue. Cureus. 2020;12(4):e7895. Published 2020 Apr 30. doi:10.7759/cureus.7895
3. Proksch E, Brandner JM, Jensen JM, The skin: an indispensable barrier. Exp Dermatol.17(12) 1063-1072. doi:10.1111/j.1600-0625.2008.00786.x
4. Elias PM. Epidermal lipids, barrier function, and desquamation. J Invest Dermatol. 1983;80(1 Suppl): 44s-49s. doi:10.1038/jid.1983.12
5. Sebastian R, Chau E, Fillmore P, et al. Epidermal aquaporin-3 is increased in the cutaneous burn wound. Burns. 2015;41(4):843-847. doi:10.1016/j.burns.2014.10.033
6. Chen YE, Fischbach MA, Belkaid Y. Skin microbiota-host interactions [published correction appears in Nature. 2018 Mar 21;555(7697):543]. Nature. 2018;553(7689):427-436. doi:10.1038/nature25177
7. Milner SM, Bhat S, Buja M, Gulati S, Poindexter BJ, Bick RJ. Expression of human beta defensin 2 in thermal injury. Burns. 2004;30(7):649-654. doi:10.1016/j.burns.2004.06.001
8. Singer M, Deutschman CS, Seymour CW, et al. The Third International Consensus Definitions for Sepsis and Septic Shock (Sepsis-3). JAMA. 2016;315(8):801-810. doi:10.1001/jama.2016.0287
9. Swain SL. T-cell subsets: Who does the polarizing? Curr Biol. 1995;5(8):849-851. doi:10.1016/S0960-9822(95)00170-9
10. Blears E, Sommerhalder C, Toliver-Kinsky T, Finnerty CC, Herndon DN. Current problems in burn immunology. Curr Probl Surg. 2020;57(6):100779. doi:10.1016/j.cpsurg.2020.100779
11. Korkmaz HI, Krijnen PAJ, Ulrich MMW, de Jong E, van Zuijlen PPM, Niessen HWM. The role of complement in the acute phase response after burns. Burns. 2017;43(7):1390-1399. doi:10.1016/j.burns.2017.03.007
12. Schwacha MG. Macrophages and post-burn immune dysfunction. Burns. 2003;29(1):1-14. doi:10.1016/s0305-4179(02)00187-0
13. Romanovsky AA. Skin temperature: its role in thermoregulation. Acta Physiol (Oxf). 2014;210(3):498-507. doi:10.1111/apha.12231
14. Zawacki BE, Spitzer KW, Mason AD Jr, Johns LA. Does increased evaporative water loss cause hypermetabolism in burned patients?. Ann Surg. 1970;171(2):236-240. doi:10.1097/00000658-197002000-00011
15. McGibbon B, Beaumont WV, Strand J, Paletta FX. Thermal regulation in patients after the healing of large deep burns. Plast Reconstr Surg. 1973;52(2):164-170. doi:10.1097/00006534-197308000-00011