ADVERTISEMENT
Imaging Surveillance of EVAR in the Era of Radiation Awareness
Abstract
lthough multiple imaging modalities are available for abdominal aortic aneurysm monitoring following EVAR, computed tomography (CT) historically has been the most commonly used modality. However, given the need for lifelong surveillance, the cumulative exposure to ionizing radiation is a concern, particularly for younger patients. As a result, other potential modalities to consider that offer the benefit of nonexposure to ionizing radiation include ultrasound, magnetic resonance angiography, and implantable pressure sensors. Given the continuous technologic advances in imaging, the body of evidence for vascular imaging is a constantly moving target. Thus in this overview we aim to provide a review of the aforementioned imaging modalities and the current state of scientific evidence, as well as to discuss the radiation concerns related to CT.
VASCULAR DISEASE MANAGEMENT 2014;11(1):E12-E20
Key words: abdominal aortic aneurysm, interventional radiology, magnetic resonance angiography (MRA), computed tomography, endovascular therapy
_______________________________
Following repair of abdominal aortic aneurysms (AAAs) with endovascular aneurysm repair (EVAR), accurate and standardized imaging is essential.1 The optimal imaging modalities and protocols may vary from patient to patient and while multiple modalities are available for monitoring, each modality has its own strengths and weaknesses.1
The goal of EVAR is to prevent AAA rupture. In order to measure treatment success, accurate AAA size must be evaluable, which is determined by AAA size stability or regression over time, and size stability is believed to indicate lower rupture risk.2 Lifelong follow-up is required, as recommended by multiple society guidelines (Society of Interventional Radiology [SIR], Cardiovascular and Interventional Radiological Society of Europe [CIRSE], and Society of Vascular Surgery).3 The guidelines put forth for post-procedural imaging by SIR suggest that any imaging modality must be able to address the following issues: (1) size measurement, (2) characterization of morphological details and placement of stent grafts, as well as (3) detection and classification of endoleaks.1 An optimal imaging modality for the surveillance of AAAs following EVAR should at the minimum include these qualities.
The risk of EVAR rupture is thought to be increased by continued perfusion of the aneurysm sac after repair (endoleak), which is one of the most common complications following EVAR.3 Endoleaks are classified according to the source of continued sac perfusion.3 A type I endoleak occurs when there is persistent sac perfusion secondary to inadequate fixation at proximal (type IA) or distal (type IB) endoprosthesis attachment sites. Type II endoleaks, the most common variant, allow perfusion of the residual AAA sac via patent branch vessels arising from the abdominal aorta such as lumbar arteries or the inferior mesenteric artery. Reversal of arterial flow through branch vessels arising from the aneurysm allows inflow into the aneurysm sac. Type III endoleaks occur when there are functional problems with devices, such as graft fabric defects or separation of modular components of the stent graft. Type IV endoleaks result from excessive graft porosity allowing continued residual sac perfusion; this type is significantly less common than in the past, largely due to manufacturer improvements in fabric design. Finally, the very rare type V endoleaks (also termed endotension) occur when there is residual post-EVAR expansion of the AAA sac without a definite demonstrable source of sac perfusion by imaging.3 In addition there are numerous other potential complications to assess for following EVAR, including vascular complications (complications of stent graft placement and issues related to vascular access), device migration (distal migration of stent graft or proximal migration of distal end) and stent-graft failure (collapse, kink, or narrowing).
Although multiple imaging modalities are available for AAA monitoring following EVAR, computed tomography (CT) has historically been the most commonly used modality.3 Given the need for lifelong surveillance, the cumulative exposure to ionizing radiation is a concern, particularly for younger patients. As a result, other potential modalities to consider that offer the benefit of nonexposure to ionizing radiation are ultrasound (US), magnetic resonance angiography (MRA), and implantable pressure sensors. Given the continuous technological advances in imaging technology, the body of evidence for vascular imaging is a constantly moving target. Thus in this overview we aim to provide a review of the aforementioned imaging modalities and the current state of scientific evidence, as well as to discuss the radiation concerns related to CT.
Computed Tomography Angiography
Computed tomography angiography (CTA) has for some time been considered the gold standard for aortic devices (Figure 1). Its advantages include excellent spatial and contrast resolution, rapid patient through-put, as well as widespread availability and reproducibility. 3
The excellent spatial resolution capabilities of CTA allow confident, reproducible evaluation of the aortic and sac dimensions, however, in addition, CTA is an important tool in monitoring stent morphology. Complications related to stent placement are readily visualized, such as stent-graft collapse (Figure 2). CTA also demonstrates excellent performance for the detection of endoleak, with sensitivity and specificity of approximately 92% and 90%, respectively.4 CTA also demonstrates excellent performance for endoleak detection compared to other modalities. Compared to conventional angiography, CTA may have higher sensitivity in detecting endoleaks following EVAR.4 Furthermore, in comparison to US, CTA is more accurate in measuring aneurysms and more sensitive for endoleak detection.3
CTA has demonstrated increased importance for AAA imaging and endoleak detection (Figure 3) following the advent of multidetector scanners and the routine availability of volumetric data sets.3 The combination of reproducibility, speed, and contrast and spatial resolution have contributed to the establishment of CTA as the preferred modality of imaging follow-up; high-resolution CT datasets allow reconstruction of thin transverse sections, multiplanar reformatted images, and accurate three-dimensional volumes.3
Although CTA offers many distinct advantages as described above, notable drawbacks include the need for contrast administration (particularly in patients with impaired renal function), cost, and radiation dosage concerns. Radiation exposure will be discussed in depth in the subsequent section, but with regard to contrast administration, contrast-induced nephropathy (CIN) is a concern. Contrast-induced nephropathy is typically defined as sudden, rapid deterioration in renal function following iodinated contrast medium without an alternative clinical explanation.5 The incidence of CIN in patients with normal renal function is under 3% but can be much higher in individuals with one or more risk factors (such as pre-existing renal function and diabetes mellitus) for CIN (12% to 50%).5-10 CIN is typically defined as an increase in serum creatinine level (≥0.5 mg/dL, ≥25%, or both), or as degradation of renal function identified by a drop in creatinine clearance or estimated glomerular filtration rate (GFR) 2-3 days following exposure to contrast agent.5,11,12
Given the high incidence of diabetes and renal insufficiency in patients with AAA, and the fairly high dose requirement of iodinated contrast with CTA, CIN is a major concern with these patients. The use of a low-osmolar contrast agent is associated with a significantly lower incidence of CIN compared with high-osmolar contrast agent in patients with baseline renal insufficiency.6,13-15 The PREDICT study further demonstrated no significant difference in the incidence of CIN in patients with diabetes and chronic kidney disease following administration of low-osmolar or isotonic contrast agents.5 The overall incidence of CIN in this study was 5.2%.5 However given these concerns overall, there is limited utility of CTA in patients with moderate to severe renal insufficiency who are not on dialysis.
The Radiation Issue
Although CTA offers several advantages as described above for AAA imaging following EVAR, both EVAR and subsequent follow-up imaging examinations expose patients to substantial amounts of radiation, with well-recognized carcinogenic risks.16 This is particularly a concern for younger patients who will be potentially undergo decades of repeated follow-up CT examinations. Thus, radiation exposure from CT scanning is an issue of patient safety that has generated significant publicity in recent times.17
Given that imaging is essential for EVAR follow-up, radiation exposure should be limited, particularly in light of carcinogenic potential, to a cumulative lifetime total of 400 millisieverts (mSv).16 A prior study demonstrated 13-fold variability between the lowest and highest effective radiation doses for the same imaging technique both within and across institutions, bringing attention to the dramatic variability of radiation exposure as well as the need for standardization.18 As a result of this high variability in radiation doses, as well as growing public awareness of the risks associated with ionizing radiation, there has been an increase in industry and legislative activities encouraging or mandating stricter oversight of imaging radiation.
For instance, beginning July 1, 2012, a California court mandated that facilities with CT and x-ray services record radiation doses on every CT study by either recording dosage within patients’ radiology reports or attaching a protocol page that includes radiation dose to the imaging report.19 Facilities conducting CT studies are required to send every CT study and protocol page listing technical factors and radiation dose to the electronic image archiving and communications system. The bill mandates the displayed dose to be annually verified by a medical physicist to ensure that displayed doses are within 20% of true measured doses, unless a facility is accredited. The bill further requires that facilities report certain adverse events to the Department of Public Health.19 For the purposes of the bill, dose radiation is to be defined as computed tomography index volume (CTDIvol) and dose length product (DLP) (Figure 4).19 Computed tomography index volume is an additional parameter for describing radiation dose, and factors in the pitch (table speed relative to gantry rotation) and represents the average radiation dose in 3 dimensions.20 DLP reflects total energy absorbed attributable to complete CT scan acquisition and is determined by the product of CTDIvol by scan length in the z-direction.20 DLP does not incorporate the tissue type exposed to radiation.
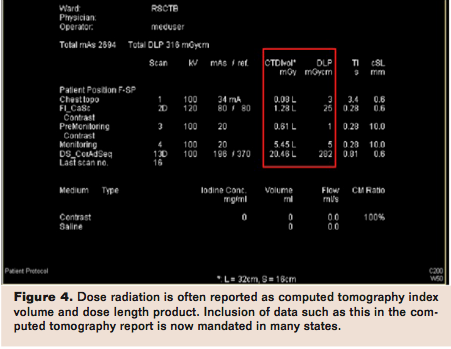
However, the so-called effective dose (E, measured in mSv), which takes into account the fact that different tissues have different sensitivities to radiation, may be estimated based on DLP by using anatomy-specific conversion factors (k).20 The advantage of effective dose is that it can be utilized to compare radiation exposures for different imaging modalities.20 To provide perspective of mean effective radiation doses outside of diagnostic imaging, natural annual environmental exposure results in 2 mSvto 3 mSv.20 CT angiography of the heart results in approximately 16 mSv of effective dose, or approximately 5 years of natural environmental exposure. The estimated average cancer induction risk in sensitive organs from cardiac CT angiography has been estimated at 0.13%, with a female to male cancer induction risk ratio of 2.6.21
Targets for reducing radiation dose include cardiac gating modes, tube current modulation, tube voltage, scan length, and pitch. For studies requiring cardiac gating, retrospective acquisition is associated with higher radiation doses because of continuous incident x-rays being generated throughout the cardiac cycle,20 thus prospective acquisition may be utilized to reduce radiation, because it allows for the x-ray beam to be turned on only during predefined phases of the cardiac cycle, and turns off the beam during all other phases.20 Tube current, a measure of the x-ray quantity of photons being generated to travel across the patient, may be modulated to produce alterations in dose as well.20 Higher tube current produces better image quality and less image noise, but at the expense of higher radiation. Tube current may be reduced by anywhere from 50% to 96% depending on the manufacturer, and overall radiation doses can be reduced by 50% to 60%.20,22
Higher tube voltage and scan length (namely the craniocaudal length along the longitudinal axis) also result in increased patient dose. Thus, lowering the patient voltage when possible, particularly in underweight and pediatric patients, is advisable.23,24 Careful planning of scan length is also advocated to limit overall radiation dose.
Finally, pitch is a dimensionless variable referring to table speed relative to gantry rotation speed; pitch is thus the amount of overlap between contiguous slabs of data.20 Pitch values are inversely proportional to radiation dose, thus pitch variation can result in significant variations in radiation dose.
With specific regard to AAA imaging, certain strategies have been described to reduce radiation dose. Maximum aneurysm diameter was used initially in the majority of studies monitoring EVAR results, but this method has been shown to be unreliable due to substantial interobserver variability.25 In an effort to reduce radiation and contrast exposure, it has been proposed to utilize serial volumetric analysis of aortic aneurysms with nonenhanced CT as the screening test for post-EVAR follow-up.26 Given the nonenhanced nature of the volumetric analysis, serial volume measurements are particularly useful for patients in whom contrast agents are contraindicated.
An additional strategy to reduce radiation exposure for AAA imaging following EVAR is to eliminate individual phases of imaging. One previously performed study assessed type II endoleaks detected by using nonenhanced and venous phase acquisitions.27 It was found that arterial phase imaging did not identify additional endoleaks in comparison to the other phases, while contributing to a mean of 36.5% of effective dose delivered. Thus, these results suggested that arterial phase imaging may not be necessary for routine endoleak detection, decreasing overall radiation exposure. On the contrary, another study found that the delayed enhanced phase imaging did not significantly increase sensitivity for endoleak detection in comparison to arterial phase imaging, although delayed phase imaging did depict low-flow endoleaks not seen on arterial phases.28 Overall, elimination of a contrast phase (possibly the arterial phase) of triple-phase CT scanning may result in considerably lower radiation dose while preserving endoleak detection rates.
The most definitive technique for eliminating radiation exposure from CT scanning is of course to use alternative modalities to follow-up EVAR repair. The modalities that will be subsequently discussed include US, MRA, and implantable pressure sensors.
Ultrasound
Ultrasound is a commonly utilized screening tool for AAA detection and may be useful for post-EVAR surveillance as well.29 Advantages of US include convenience, portability, safety, and relative low cost, while downsides include operator dependence, with scan quality and protocols varying greatly between institutions.29,30 Obesity and overlying bowel gas offer additional potential obstacles to accurate AAA imaging with US.
Previously performed studies have demonstrated excellent correlation between CT and ultrasound for aneurysm sac size after EVAR.31,32 However, both studies demonstrated limited agreement between actual measurements of AAA diameter between US and CT, with US underestimating AAA diameter compared to CT between 1.8 and 2.9 mm.31,32 In addition, for AAA diameter change, one of these studies showed that in 23% of cases of AAA repair, US demonstrated diameter change that would have influenced patient management, while CT did not demonstrate changes in these cases.31 Finally, while US demonstrates excellent specificity for endoleak detection (91% to 93%), sensitivity is limited (66% to 69%) (Figure 5).33,34 While US may have some role for AAA follow-up following EVAR, one reason CT has persisted in the follow-up regimen for EVAR is the demand for precise, reproducible aneurysm size measurement.29
Advances in US technology beyond standard US imaging offer the potential to improve US for AAA follow-up. The role of conventional US for imaging is limited by capabilities of traditional color and spectral Doppler imaging.35 While Doppler imaging may provide valuable directional flow information, its ability to detect blood flow at the perfusion level is limited.35
The use of microbubble contrast agents remedies this situation by facilitating US capability of detecting endoleak.29,34,36-39 Microbubble agents enhance Doppler signal from blood, while specialized US techniques serve to suppress signals from background tissue while enhancing sensitivity to Doppler signals from microbubbles within the blood pool.35
Microbubble contrast agents are composed of tiny bubbles of injectable gas within a supporting shell that functions to counter the effects of surface tension and thereby increasing their longevity (Figure 6).40 The effectiveness of microbubble contrast agents is due to their dynamic response to the application of an ultrasound field.40 As a result of their compressibility they undergo volumetric oscillation (pulsation) and thereby scatter much more energy than rigid spheres of the same size would.40
The evidence for use of such agents includes ease of use, contribution to patient care, and favorable safety profile.41 Microbubble agents are not nephrotoxic and may be used in patients with any degree of renal function. Furthermore, their use does not result in damaging ionizing radiation, an important factor for patients undergoing repeated examinations.35 One European retrospective review of microbubble contrast agents in over 23,000 patients reported only 2 serious adverse events and no deaths.42 While microembolism and tissue heating are theoretical side effects, single microbubble contrast agents do not present a significant risk, and furthermore albumin-shelled agents do not coalesce to form larger particles that would potentially block capillaries.40,43 Furthermore, the risk of heating secondary to contrast US is likely minimal.40
As stated, the actual performance of contrast-enhanced US involves suppression of signal from background tissue, leaving only signal from microbubbles.35 This is most commonly accomplished by pulse inversion whereby two signals are sent down a single scan line and the second is a mirror image of the first. Echoes from both pulses are collected by the US transducer and summed. Linear reflectors like normal tissue produce no net signal; however nonlinear reflectors, like microbubbles, produce echoes that are asymmetric and do not sum to zero, resulting in preferential detection of bubbles in this fashion.35 This phenomenon forms the basis behind the mechanism of contrast-enhanced microbubble US.
With regards to the application of contrast-enhanced US to AAA imaging, early studies demonstrated low sensitivity, and later studies with more recent agents incorporating tissue harmonic imaging have demonstrated better results, with contrast-enhanced US occasionally showing endoleaks that could not have been seen with CTA or conventional US.29 This suggests that contrast-enhanced US could be utilized as a problem-solving tool in situations of suspected endotension, and also possibly expand the routine usage of US for AAA follow-up after EVAR.29
Magnetic Resonance Angiography
Magnetic resonance angiography (MRA) with gadolinium is an additional modality that may be utilized for follow-up imaging in evaluating AAA after EVAR (Figure 7). A primary advantage of MRA in comparison to CTA is the avoidance of ionizing radiation, which is a significant advantage given the number of serial annual examinations these patients undergo. Conversely, disadvantages to MRA include cost, relative inaccessibility compared to CTA, longer scan times and effects on patient comfort, lower spatial resolution, as well as noncompatibility with cardiac and neural devices. In addition, performance is dependent on composition of stent-grafts, which may produce artifact. Nitinol-based (nickel and titanium) stents are typically more suitable for MRA and allow visualization of stent lumen and adjacent structures. However elgiloy stents (cobalt, chromium, and nickel) can obscure vessel lumen and stainless stents may cause extensive artifact and provide poor local visualization, rendering studies nondiagnostic.29
The current body of evidence for MRA is limited, but despite the aforementioned downsides, a small number of studies have demonstrated comparative benefits of MRA in post-EVAR imaging. In several studies with small patient numbers with predominantly nitinol stents, MRA was seen to be at least as sensitive as CTA, and in some cases demonstrated endoleaks not seen with CTA.44-49 In one case report, patients with enlarging aneurysm sacs were suspected of having endotension, but were shown by subsequent MRA to have type II endoleaks not initially identified with CTA.50
Although most MRA studies rely on dynamic gadolinium-enhanced three-dimensional MRA, newer MRA technologies such as time-resolved MRA may allow better endoleak type characterization by demonstrating temporal evolution of contrast material in the aneurysm sac, analogous to conventional angiography.29,51,52 Thus, with the further development of newer MRA technologies for vascular imaging, MRA may continue to play a larger role in post-EVAR imaging for AAA, particularly when nitinol-based stents are utilized.
Implantable Pressure Sensors
Remote wireless pressure sensor monitoring is a novel technology with potential benefit in the setting of surveillance of endovascular grafts.53 Although radiologic monitoring is essential to care of patients following EVAR, measuring aneurysm sac pressure is linked to effective therapeutic aneurysm sac exclusion.53,54 Of importance, these sensors monitor sac pressure, in theory an ideal metric of endovascular graft efficacy, whereas changes in aneurysm size and sac contrast enhancement serve as indirect criteria for ongoing aneurysm sac pressurization.53
Clinical feasibility trials have confirmed device durability and efficacy and show that reduction in aneurysm sac pressurization ratio of less than 30% (sac pulse pressure remains over 70% of systemic pulse pressure) following endovascular graft deployment is compatible with type I or type III endoleak (sensitivity 94%, specificity 80%).55 Of note, the United States Food and Drug Administration (FDA) has approved device utilization for intraoperative endoleak detection in the thoracic aorta; however, FDA approval excludes longer-term surveillance, although this represents an important potential use for this technology.53
As the implantable pressure sensor is a newer technology, data is still currently being accumulated, but implantation of these devices offers the potential to provide useful information regarding endoleak following EVAR and may help guide clinical therapy and improving outcomes. Specifically if validated, this technology may reduce the frequency of scheduled follow-up imaging, and also may prompt urgent imaging if an abnormality is detected.53
Cost-Effectiveness
An additional potential consideration of imaging modality selection is the differential costs between procedures. A prior economic analysis across radiologic modalities in a tertiary academic setting demonstrated individual examination costs as follows: $50.28 for US, $112.32 for CT, $266.96 for MR imaging, and $692.69 for interventional radiology (the category that would be applicable to conventional angiography).56 The costs per technical relative value unit (RVU) for US, CT, MR imaging, and interventional radiology were $28.74, $20.95, $17.69, and $89.03, respectively.56 These findings indicate that the method of quantifying cost results in different cost values per modality. A separate study assessing patients with peripheral arterial disease demonstrated that US had higher cost effectiveness at a lower cost per quality-adjusted life year over MR, CT, and angiography.57 Thus especially in patients undergoing multiple serial examinations, the economic considerations could also play a contributing role in determining the choice of imaging modality; they should be balanced with physical risks to the patient, as well as by the drive to select the modality that would provide the greatest benefit to the patient’s care.
Conclusion
The quality of published studies involving CTA and alternative modalities is variable and undergoing constant additions as newer studies are performed. Much of the data is likely outdated and updated studies are warranted to incorporate both new modalities as well as upgrades to currently existing imaging technologies. Although CTA is currently the gold standard for imaging for post-EVAR follow-up for AAA, MRA offers an additional modality with excellent sensitivity and specificity (especially in the setting of nitinol stents), and US offers superior cost and safety profiles. Direct intrasac pressure measurement tools are currently undergoing active testing and offer an exciting potential additional tool for the future.
Currently common time points for performing CTA after EVAR are 1 month, 6 months, 12 months, and annually thereafter.58 However, it was found that experienced centers frequently discard the 6-month post-EVAR scan for noncomplicated cases, and also that experienced centers were more likely to use US as a follow-up modality.58 Thus pursuing a similar imaging protocol following EVAR would be in line with what many centers appear to be performing currently after EVAR. While MRA is not as routinely performed at many centers, we believe that in certain cases MRA offers the possibility of use as an additional problem-solving tool.
The clinical decision-making in real practice is of course complex and highly dependent upon the set of particular patient circumstances (such as the presence of vascular calcifications in diabetic patients with impaired renal function). Finally, most analyses of performance do not consider relative costs of each imaging tests, which in reality have significant practical importance. The current state of practice is sure to be modified as time progresses with the evolution of new techniques, as well as through the publication of new results.
Editor’s Note: Disclosure: The authors have completed and returned the ICMJE Form for Disclosure of Potential Conflicts of Interest. The authors report no conflicts of interest related to the content herein.
Manuscript received June 25, 2013; provisional acceptance given August 5, 2013; final version accepted August 21, 2013.
Address for correspondence: John M. Moriarty, MD, UCLA Medical Center, David Geffen School of Medicine at UCLA, Department of Radiology, Peter V. Ueberroth Bldg, Suite 3371, Los Angeles, CA 90095, United States. Email: JMoriarty@mednet.ucla.edu
References
- Geller SC. Imaging guidelines for abdominal aortic aneurysm repair with endovascular stent grafts. J Vasc Interv Radiol. 2003;14(9 Pt 2):S263-S264.
- Schanzer A, Greenberg RK, Hevelone N, et al. Predictors of abdominal aortic aneurysm sac enlargement after endovascular repair. Circulation. 2011;123(24):2848-2855.
- Walker TG, Kalva SP, Yeddula K, et al. Clinical practice guidelines for endovascular abdominal aortic aneurysm repair: written by the Standards of Practice Committee for the Society of Interventional Radiology and endorsed by the Cardiovascular and Interventional Radiological Society of Europe and the Canadian Interventional Radiology Association. J Vasc Interv Radiol. 2010;21(11):1632-1655.
- Armerding MD, Rubin GD, Beaulieu CF, et al. Aortic aneurysmal disease: assessment of stent-graft treatment-CT versus conventional angiography. Radiology. 2000;215(1):138-146.
- Kuhn MJ, Chen N, Sahani DV, et al. The PREDICT study: a randomized double-blind comparison of contrast-induced nephropathy after low- or isoosmolar contrast agent exposure. AJR Am J Roentgenol. 2008 Jul;191(1):151-157.
- Lautin EM, Freeman NJ, Schoenfeld AH, et al. Radiocontrast-associated renal dysfunction: incidence and risk factors. AJR Am J Roentgenol.1991 Jul;157(1):49-58.
- McCullough PA, Wolyn R, Rocher LL, Levin RN, O’Neill WW. Acute renal failure after coronary intervention: incidence, risk factors, and relationship to mortality. Am J Med. 1997;103(5):368-375.
- Parfrey PS, Griffiths SM, Barrett BJ, et al. Contrast material-induced renal failure in patients with diabetes mellitus, renal insufficiency, or both. A prospective controlled study. New Engl J Med. 1989;320(3):143-149.
- Rihal CS, Textor SC, Grill DE, et al. Incidence and prognostic importance of acute renal failure after percutaneous coronary intervention. Circulation. 2002;105(19):2259-2264.
- Weisberg LS, Kurnik PB, Kurnik BR. Risk of radiocontrast nephropathy in patients with and without diabetes mellitus. Kidney Int. 1994;45(1):259-265.
- Thomsen HS. Guidelines for contrast media from the European Society of Urogenital Radiology. AJR Am J Roentgenol. 2003;181(6):1463-1471.
- McCullough PA, Sandberg KR. Epidemiology of contrast-induced nephropathy. Rev Cardiovasc Med. 2003;4 Suppl 5:S3-S9.
- Barrett BJ, Carlisle EJ. Metaanalysis of the relative nephrotoxicity of high- and low-osmolality iodinated contrast media. Radiology. 1993;188(1):171-178.
- Rudnick MR, Goldfarb S, Wexler L, et al. Nephrotoxicity of ionic and nonionic contrast media in 1196 patients: a randomized trial. The Iohexol Cooperative Study. Kidney Int. 1995;47(1):254-261.
- Taliercio CP, Vlietstra RE, Ilstrup DM, et al. A randomized comparison of the nephrotoxicity of iopamidol and diatrizoate in high risk patients undergoing cardiac angiography. J Am Coll Cardiol. 1991;17(2):384-390.
- Jones C, Badger SA, Boyd CS, Soong CV. The impact of radiation dose exposure during endovascular aneurysm repair on patient safety. J Vasc Surg. 2010;52(2):298-302.
- Rabin RC. With rise in radiation exposure, experts urge caution on tests. The New York Times. 2007. https://www.nytimes.com/2007/06/19/health/19cons.html?_r=0.
- Smith-Bindman R, Lipson J, Marcus R, et al. Radiation dose associated with common computed tomography examinations and the associated lifetime attributable risk of cancer. Arch Intern Med. 2009;169(22):2078-2086.
- Cal SB 1237 (2010). https://www.leginfo.ca.gov/pub/09-10/bill/sen/sb_1201-1250/sb_1237_bill_20100929_chaptered.html.
- Vorobiof G, Achenbach S, Narula J. Minimizing radiation dose for coronary CT angiography. Cardiology Clinics. 2012;30(1):9-17.
- Huda W, Schoepf UJ, Abro JA, Mah E, Costello P. Radiation-related cancer risks in a clinical patient population undergoing cardiac CT. AJR Am J Roentgenol. 2011;196(2):W159-W165.
- Blanke P, Bulla S, Baumann T, et al. Thoracic aorta: prospective electrocardiographically triggered CT angiography with dual-source CT--feasibility, image quality, and dose reduction. Radiology. 2010;255(1):207-217.
- Herzog C, Mulvihill DM, Nguyen SA, et al. Pediatric cardiovascular CT angiography: radiation dose reduction using automatic anatomic tube current modulation. AJR Am J Roentgenol. 2008;190(5):1232-1240.
- Suess C, Chen X. Dose optimization in pediatric CT: current technology and future innovations. Pediatr Radiol. 2002;32(10):729-734.
- Dugas A, Therasse E, Kauffmann C, et al. Reproducibility of abdominal aortic aneurysm diameter measurement and growth evaluation on axial and multiplanar computed tomography reformations. Cardiovasc Intervent Radiol. 2012;35(4):779-787.
- Bley TA, Chase PJ, Reeder SB, et al. Endovascular abdominal aortic aneurysm repair: nonenhanced volumetric CT for follow-up. Radiology. 2009;253(1):253-262.
- Macari M, Chandarana H, Schmidt B, Lee J, Lamparello P, Babb J. Abdominal aortic aneurysm: can the arterial phase at CT evaluation after endovascular repair be eliminated to reduce radiation dose? Radiology. 2006;241(3):908-914.
- Iezzi R, Cotroneo AR, Filippone A, et al. Multidetector CT in abdominal aortic aneurysm treated with endovascular repair: are unenhanced and delayed phase enhanced images effective for endoleak detection? Radiology. 2006;241(3):915-921.
- Stavropoulos SW, Charagundla SR. Imaging techniques for detection and management of endoleaks after endovascular aortic aneurysm repair. Radiology. 2007 Jun;243(3):641-55. PubMed PMID: 17517926. Epub 2007/05/23. eng.
- Sato DT, Goff CD, Gregory RT, et al. Endoleak after aortic stent graft repair: diagnosis by color duplex ultrasound scan versus computed tomography scan. J Vasc Surg. 1998;28(4):657-663.
- Elkouri S, Panneton JM, Andrews JC, et al. Computed tomography and ultrasound in follow-up of patients after endovascular repair of abdominal aortic aneurysm. Ann Vasc Surg. 2004;18(3):271-279.
- Bargellini I, Cioni R, Napoli V, Petruzzi P, Vignali C, Cicorelli A, et al. Ultrasonographic surveillance with selective CTA after endovascular repair of abdominal aortic aneurysm. J Endovasc Ther. 2009 Feb;16(1):93-104.
- Ashoke R, Brown LC, Rodway A, et al. Color duplex ultrasonography is insensitive for the detection of endoleak after aortic endografting: a systematic review. J Endovasc Ther. 2005;12(3):297-305.
- Sun Z. Diagnostic value of color duplex ultrasonography in the follow-up of endovascular repair of abdominal aortic aneurysm. J Vasc Interv Radiol. 2006;17(5):759-764.
- Wilson SR, Greenbaum LD, Goldberg BB. Contrast-enhanced ultrasound: what is the evidence and what are the obstacles? AJR Am J Roentgenol. 2009;193(1):55-60.
- Bendick PJ, Bove PG, Long GW, Zelenock GB, Brown OW, Shanley CJ. Efficacy of ultrasound scan contrast agents in the noninvasive follow-up of aortic stent grafts. J Vasc Surg. 2003;37(2):381-385.
- Heilberger P, Schunn C, Ritter W, Weber S, Raithel D. Postoperative color flow duplex scanning in aortic endografting. J Endovasc Surg. 1997;4(3):262-271.
- McWilliams RG, Martin J, White D, et al. Detection of endoleak with enhanced ultrasound imaging: comparison with biphasic computed tomography. J Endovasc Ther. 2002;9(2):170-179.
- Napoli V, Bargellini I, Sardella SG, et al. Abdominal aortic aneurysm: contrast-enhanced US for missed endoleaks after endoluminal repair. Radiology. 2004;233(1):217-225.
- Stride E, Saffari N. Microbubble ultrasound contrast agents: a review. Proc Inst Mech Eng H. 2003;217(6):429-447.
- Main ML, Ryan AC, Davis TE, Albano MP, Kusnetzky LL, Hibberd M. Acute mortality in hospitalized patients undergoing echocardiography with and without an ultrasound contrast agent (multicenter registry results in 4,300,966 consecutive patients). Am J Cardiol. 2008;102(12):1742-1746.
- Piscaglia F, Bolondi L; Italian Society for Ultrasound in Medicine and Biology (SIUMB) Study Group on Ultrasound Contrast Agents. The safety of Sonovue in abdominal applications: retrospective analysis of 23188 investigations. Ultrasound Med Biol. 2006;32(9):1369-1375.
- Nyborg WL. Biological effects of ultrasound: development of safety guidelines. Part II: general review. Ultrasound Med Biol. 2001;27(3):301-333.
- Ayuso JR, de Caralt TM, Pages M, et al. MRA is useful as a follow-up technique after endovascular repair of aortic aneurysms with nitinol endoprostheses. J Magn Reson Imaging. 2004;20(5):803-810.
- Cejna M, Loewe C, Schoder M, et al. MR angiography vs CT angiography in the follow-up of nitinol stent grafts in endoluminally treated aortic aneurysms. Eur Radiol. 2002;12(10):2443-2450.
- Haulon S, Willoteaux S, Koussa M, Gaxotte V, Beregi JP, Warembourg H. Diagnosis and treatment of type II endoleak after stent placement for exclusion of an abdominal aortic aneurysm. Ann Vasc Surg. 2001;15(2):148-154.
- Insko EK, Kulzer LM, Fairman RM, Carpenter JP, Stavropoulos SW. MR imaging for the detection of endoleaks in recipients of abdominal aortic stent-grafts with low magnetic susceptibility. Acad Radiol. 2003 May;10(5):509-513.
- Pitton MB, Schweitzer H, Herber S, et al. MRI versus helical CT for endoleak detection after endovascular aneurysm repair. AJR Am J Roentgenol. 2005;185(5):1275-1281.
- van der Laan MJ, Bartels LW, Viergever MA, Blankensteijn JD. Computed tomography versus magnetic resonance imaging of endoleaks after EVAR. Eur J Vasc Endovasc Surg. 2006;32(4):361-365.
- Wicky S, Fan CM, Geller SC, Greenfield A, Santilli J, Waltman AC. MR angiography of endoleak with inconclusive concomitant CT angiography. AJR Am J Roentgenol. 2003;181(3):736-738.
- Lookstein RA, Goldman J, Pukin L, Marin ML. Time-resolved magnetic resonance angiography as a noninvasive method to characterize endoleaks: initial results compared with conventional angiography. J Vasc Surg. 2004;39(1):27-33.
- van der Laan MJ, Bakker CJ, Blankensteijn JD, Bartels LW. Dynamic CE-MRA for endoleak classification after endovascular aneurysm repair. Eur J Vasc Endovasc Surg. 2006 Feb;31(2):130-135.
- Parsa CJ, Daneshmand MA, Lima B, Balsara K, McCann RL, Hughes GC. Utility of remote wireless pressure sensing for endovascular leak detection after endovascular thoracic aneurysm repair. Ann Thorac Surg. 2010;89(2):446-452.
- Baum RA, Carpenter JP, Cope C, et al. Aneurysm sac pressure measurements after endovascular repair of abdominal aortic aneurysms. J Vasc Surg. 2001;33(1):32-41.
- Ohki T, Ouriel K, Silveira PG, et al. Initial results of wireless pressure sensing for endovascular aneurysm repair: the APEX Trial--Acute Pressure Measurement to Confirm Aneurysm Sac EXclusion. J Vasc Surg. 2007;45(2):236-242.
- Saini S, Seltzer SE, Bramson RT, et al. Technical cost of radiologic examinations: analysis across imaging modalities. Radiology. 2000;216(1):269-272.
- Collins R, Cranny G, Burch J, et al. A systematic review of duplex ultrasound, magnetic resonance angiography and computed tomography angiography for the diagnosis and assessment of symptomatic, lower limb peripheral arterial disease. Health Technol Assess. 2007;11(20):iii-iv, xi-xiii, 1-184.
- Uthoff H, Pena C, Katzen BT, et al. Current clinical practice in postoperative endovascular aneurysm repair imaging surveillance. J Vasc Interv Radiol. 2012;23(9):1152-1159.