ADVERTISEMENT
The Current State of Endovascular Intervention for Peripheral Arterial Disease
Abstract
The rising incidence of peripheral arterial disease (PAD) has resulted in a concomitant increase in complications such as critical limb ischemia and lower-extremity amputation. Although a full spectrum of therapies exists for femoropopliteal PAD, there is not yet a well-defined consensus on the role of a specific type of endovascular modality that is best suited for treatment. Although percutaneous transluminal angioplasty (PTA) is commonly used for revascularization in PAD due to high initial success rates of around 90%, long-term patency is a concern. Studies comparing drug-eluting balloons to PTA have demonstrated encouraging results with respect to primary patency but inconsistent results from the perspective of freedom from target lesion revascularization and adverse events. While stent placement has primarily been utilized as “bail-out” therapy for PTA with complications, newer generation drug-eluting stents including sirolimus-eluting and everolimus-eluting stents have shown favorable outcomes, suggesting an increasing role for this form of percutaneous revascularization. Although a diverse array of atherectomy devices is available, the role of these devices has yet to be clearly defined due to lack of high-quality studies that are appropriately powered to evaluate for long-term clinical outcomes. Despite this, the continued study of these modalities complemented by refinements in technique for new devices foreshadows a bright future for new endovascular approaches in the treatment of PAD.
VASCULAR DISEASE MANAGEMENT 2015;12(10):E190-E203
Key words: peripheral vascular disease, drug-eluting stents, stenting, drug-eluting balloons, atherectomy, critical limb ischemia
_____________________
As life expectancy continues to increase, comorbidities that contribute to metabolic syndrome, including diabetes mellitus, have become increasingly prevalent. These comorbidities serve as risk factors for the development of peripheral arterial disease (PAD), resulting in complications such as lifestyle-limiting claudication and critical limb ischemia (CLI). More than 200 million people are affected by PAD worldwide, with nearly 27 million of those individuals residing in North America and Europe.1,2 The greater prevalence of PAD has caused a concomitant increase in lower-extremity amputation in the United States, with rise in rate from 19 to 30 amputations per 100,000 person-years over the past 2 decades.2,3
Treatment for PAD includes pharmacotherapy as well as endovascular and surgical revascularization. Percutaneous vascular intervention (PVI) is an effective treatment option, particularly for patients with type A or B TransAtlantic Inter-Society Consensus (TASC II) disease, those with favorable anatomy to a percutaneous approach, and those who are otherwise poor surgical candidates.2,4 While a number of endovascular modalities are available, a clear consensus on the superiority of a particular type of treatment has not yet emerged. We review the current data on endovascular therapies for PAD.
Balloon Angioplasty
The repeatability, low complication rate, and less invasive nature of percutaneous transluminal angioplasty (PTA) render this modality suitable for revascularization for many lesions, especially those that are not heavily calcified.5,6 The introduction of long balloons allows for widespread use of PTA including for long lesions and patients with diffuse disease, especially given the often multilevel and multivessel nature of PAD. Initial success rates are in the 90% range for PTA of the superficial femoral artery (SFA) and infrapopliteal arteries.7-9 However, long-term vessel patency is a concern, with restenosis occurring in greater than 60% of cases at 12-month follow-up.9-12 Consequently, reintervention is often required to restore patency. Reintervention for restenosis is associated with worsened surgical outcomes as well as increased morbidity and mortality, in part due to the high level of comorbidity in the PAD patient population.13-15
Drug-Eluting Balloons
Drug-eluting balloons (DEBs) are effective in the coronary vasculature, particularly in small arteries and bifurcation disease.16 Several studies have established the benefit of DEB compared to PTA in primary patency for femoropopliteal disease.17-19 The LEVANT I trial demonstrated the superiority of paclitaxel-coated balloons (PCB) over PTA, with 58% less lumen loss at 6 months and similar safety profile to PTA.19 Lower rates of target lesion revascularization (TLR) have also been reported with PCB use.17,18
The 5-year results of the THUNDER trial demonstrated lower rates of TLR with PCB as compared to PTA (21% vs 56%; P=.0005) without significant differences in adverse events, amputation rate, and death.20 Similar positive results with PCB have been reported in studies of patients undergoing PVI in the infrapopliteal territory.21,22 The DEBATE-BTK trial showed striking reductions in 1-year restenosis (27% in DCB vs 74% in PTA; P<.001) and TLR (18% in PCB vs 43% in PTA; P=.002) in diabetic patients with high Rutherford-Becker class (greater than IV).22 However, the IN.PACT DEEP trial reported that the Amphirion PCB (Medtronic) was noninferior to PTA in terms of TLR and late lumen loss, but with a trend toward increased complications including a higher rate of major amputation at 12 months (8.8% vs 3.6%; P=.080), resulting in withdrawal of the device from the market.23
It has been difficult to ascertain why poor outcomes were encountered in this trial, although excellent PTA technique, inconsistent post-procedure wound care, and the method in which IN.PACT Amphirion PCBs are constructed leading to inconsistent paclitaxel distribution may be responsible for the lack of treatment effect.23,24 The LEVANT II trial, a highly powered 54-site randomized control trial of 476 patients with symptomatic intermittent claudication or ischemic pain while at rest and angiographically significant atherosclerosis, showed greater primary patency rates for those who had undergone angioplasty with a PCB as opposed to PTA (65.2% vs 52.6%, P=.02) with noninferior safety outcomes at 12 months.25 However, TLR and functional outcomes such as rates of reintervention, thrombosis, amputation and were similar among groups.25 In order to better clarify the role of DEB in clinical practice, forthcoming studies should explore head-to-head comparisons of DEB vs stenting and atherectomy modalities.
Stenting
Although stent implantation has been utilized as a “bail-out” treatment for some patients with residual stenosis, significant recoil, and flow-limiting dissection after PTA, they are also used as primary therapy with the hope of reducing the risk of restenosis.26 Self-expanding nitinol bare-metal stents (BMS) improve outcomes compared to PTA in femoropopliteal arterial disease, even in complicated patients with multiple comorbidities.27-30 A study of 104 patients with femoropopliteal disease demonstrated the superiority of primary or secondary self-expanding nitinol BMS placement compared to PTA in terms of restenosis (49.2% vs 74.3%; P=.028) at 2 years.29 The STROLL trial, a single-arm, multicenter study of 250 patients, revealed excellent results with the use of self-expanding nitinol BMS for SFA disease (mean age 67.7±10.3 years, 47.2% with diabetes mellitus, mean lesion length 77.3±35.3 mm) with 100% freedom from 30-day major adverse events (death, index limb amputation, and clinically driven TLR) with sustained results at 12-month follow-up.31 Moreover, primary stenting improved long-term clinical outcomes such as ankle-brachial index (ABI) values and treadmill walking capacity.29,31 Reassuring results have also been obtained in studies involving infrapopliteal stenting. A meta-analysis of patients undergoing both balloon-expandable and self-expandable BMS for infrapopliteal disease showed satisfactory angiographic results, patency, and clinical outcomes over a 12-month period.32
By utilizing graft material to prevent intimal hyperplasia and reduce long-term restenosis, covered stents were developed with the prospect of improving pre-existing stent technology. Although early studies of polyester-covered stent-grafts yielded poor results because implantation was associated with low patency rates and a significant post-procedural inflammatory response, the use of expanded polytetrafluoroethylene (ePTFE)-covered stents has shown favorable outcomes.33-36 The Viabahn Endoprosthesis (W. L. Gore & Associates) is a non-heparin-bonded endoluminal graft with an ePTFE lining and external nitinol support that was FDA approved in 2005 for SFA implantation. A number of investigators have studied this device and have determined primary and secondary patency rates ranging from 49% to 78.7% and 61% to 93.4% respectively.37-40 In comparison to PTA, use of the Viabahn stent-graft has shown superior patency outcomes at 12-month follow-up (65% vs 40%; P=.0003), with an additional benefit for lesions ≥3cm in length.41 Despite these results, 36-month data from the VIBRANT trial comparing Viabahn Endoprosthesis to bare nitinol stent implantation for severe SFA disease (TASC C+D) revealed no significant difference in primary or secondary patency rates between groups (24.2% vs 25.9%; P=.392 and 89.3% vs 79.5%; P=.304 respectively).42 Nevertheless, the Viabahn stent-graft fractured less and both groups equally showed improvement in hemodynamic and other quality measures.42 To account for concerns regarding edge restenosis and stent thrombosis, the FDA approved a version of the Viabahn device with a heparin-bonded surface in 2007. Initial nonrandomized studies on the technology demonstrated improved biocompatibility by affecting protein absorption and thromboresistance, therefore extending patency time.43,44 The VIASTAR trial, which evaluated 24-month outcomes of ePTFE-covered stents to BMS in patients with symptomatic peripheral arterial disease (PAD), found that patients with long femoropopliteal lesions demonstrated significantly higher primary patency rates in the heparin-bonded covered stent group (63.1% vs 41.2%; P=.04), but without a significant effect on freedom from TLR (80.0% vs 61.9%; P=.13).45
The aSpire stent (LeMaitre Vascular) is another covered-stent platform that is comprised of a nitinol double-spiral body, allowing for better contour to the vessel lumen and reduced obstruction of arterial side-branches. Use of the aSpire stent has been shown to have acceptable long-term results with primary cumulative patency rate of 60.6% with a primary assisted patency of 70.2% at 33 months in patients with long-segment SFA occlusive disease.46 However, further experience with aSpire stenting reveals that a number of reinterventions are often necessary to obtain optimal patency results, suggesting the need for technologic and pharmacologic improvements.47
The SIROCCO trial, which was the first double-blinded, randomized controlled trial comparing sirolimus-eluting stents (SES) to BMS in the femoropopliteal distribution, along with a follow-up study evaluating long-term outcomes found similar rates of restenosis, TLR, ABI, and claudication symptoms between SES and BMS.48,49 The unexpectedly low restenosis rate with BMS and suboptimal drug elution of SES may account for the failure to demonstrate superiority of SES in this trial.49
The Zilver PTX trial demonstrated a higher 5-year primary patency rate with paclitaxel-eluting stents compared with PTA or provisional BMS for SFA disease (66.4% vs. 43.4%).50-52 Enhancements pursued in this trial, as compared to the SIROCCO trial, included higher antiproliferative agent dosing density, lack of binding polymer on the stent to reduce the risk of delamination from repeated mechanical stress, and importantly, PTA with subsequent “bail-out” stenting as a choice for the control arm.51 Criticisms of this trial included the relatively short lesions lengths. Other stent platforms, including the Dynalink nitinol self-expanding stent (Abbott Vascular), which uses an everolimus-eluting stent (EES) with a longer elution profile, have also established favorable outcomes and clinical improvements for femoropopliteal arterial disease, although an even longer drug-elution profile may be necessary for patients with longer lesions given the high number of restenosis events encountered in this study after 6 months.51,53
Data from clinical trials reveal that the treatment of infrapopliteal disease with drug-eluting stents (DES) leads to significantly greater patency rates when compared to PTA or BMS.54 The ACHILLES trial, which included 200 patients with infrapopliteal disease, demonstrated higher primary patency rates with SES (80.6% vs 58.1%; P=.006) and lower TLR rates (10% vs 16.5%; P=.26) compared with PTA.55 The YUKON-BTK and DESTINY trials have shown superiority in primary lumen patency at 12-month follow-up of SES and EES as compared to BMS, respectively (80.6% in SES vs 55.6% in BMS; P<.05 and 85.2% in EES vs 54.4% in BMS; P<.05).56,57 A review of 18 nonrandomized studies comparing SES to BMS show superiority of SES in the prevention of restenosis (P<.001) and also show superiority of SES to paclitaxel-eluting stents with regard to primary patency and need for revascularization at 12 months (P=.014).32
A single prospective study evaluating EES to PTA with provisional BMS placement in lesions >4.5 cm showed higher primary patency with EES vs PTA/BMS (29.7% vs 20.6%; P<.0001) as well as improved event-free survival (hazard ratio 2.2, 95% confidence interval 1.2-4.1, P=.015), demonstrating that patients with longer lesions can also benefit from EES.58 However, a retrospective dual-center study comparing DEB to DES for 228 patients of all Rutherford-Becker classes with long lesions (>10 cm) found similar rates of binary restenosis (23.9% vs 30.4%; P=.319) and TLR (15.6% vs 19.0%; P=.543).59
Atherectomy
Several types of atherectomy devices are available for plaque modification and debulking (Table 1). Excisional atherectomy utilizes an intra-arterial blade to remove plaque from the arterial wall (Figure 1). An advantage to this approach as compared to other endovascular interventions includes the ability to maximize luminal gain by directing treatment toward the target lesion, even when those lesions are re-stenotic or excessively calcified.60-62 The initial outcomes from studies that employed atherectomy were encouraging but were not considerably different than PTA, thus limiting use of this technology early on.63 Subsequent studies of excisional atherectomy have demonstrated variable primary patency rates at 12 months (43% to 84%) with even poorer rates in re-stenotic lesions.64-66 Moreover, worsening patency and restenosis rates have been observed in patients with more significant disease (TASC C+D). A retrospective analysis of patients with symptomatic lower-limb PAD undergoing atherectomy with the TurboHawk atherectomy device (Medtronic/Covidien) by a single clinician on 47 vessels demonstrated 6- and 12-month patency rates of 72.6% and 58.9%, respectively.67 When atherectomy was assisted by other measures, the primary patency rate was 93.2% and 74.6% at 6 months and 12 months, respectively.67 Notably, patients in the earlier cohort had a patency rate of 41.3% as compared to 82.9% for patients in the latter cohort (P=.001), indicating that there may be a learning curve associated with usage of directional atherectomy.67 Furthermore, the initial cases included aggressive atherectomy over longer segments whereas patients near the end of the series were treated more conservatively over shorter segments.
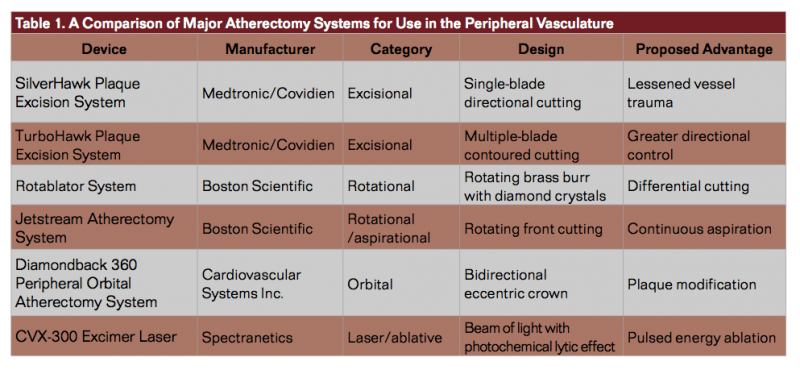
Rotational atherectomy involves the use of a concentrically spinning burr in association with a drive shaft and turbine.68 The proposed advantage of rotational atherectomy is “differential cutting,” where the device atherectomizes the diseased inelastic plaque as opposed to healthy elastic tissue, as well as the ability to better advance the burr through narrowed and calcified vessels. Although high initial success rates have been achieved with the use of rotational atherectomy vs PTA in the coronary circulation (89% vs 80%; P=.0019), TLR is increased (42.4% vs 31.9%; P=.013).69 Follow-up multicenter data demonstrate similar lack of benefit in the prevention of restenosis, limiting use of rotational atherectomy as pretreatment for heavily calcified lesions prior to stenting.70 Currently, rotational atherectomy with devices like the Rotablator (Boston Scientific) is rarely used for PVI. Improvements to rotational atherectomy technology, as developed for the Jetstream Atherectomy System (Boston Scientific), allow for thrombectomy along with rotational atherectomy from the same catheter as well as continual active aspiration for the removal of debris (Figure 2).71 Although a single-center study utilizing this system revealed 99% device success with clinically driven TLR rate of 26% at 1 year, a large review evaluating this system along with a similar device showed a combined distal embolization rate of 22% vs 1.9% with excisional atherectomy, vs 0.9% with PTA alone, and 0.7% with PTA followed by stenting.72,73
Operating with a crown similar to the rotational atherectomy burr, orbital atherectomy (Diamondback 360° Peripheral Orbital Atherectomy System; Cardiovascular Systems, Inc.) employs an orbiting action to modify plaque as well as increase lumen size (Figure 3).74-77 Prospective, multicenter data evaluating the use of orbital atherectomy demonstrated that shorter spin times and smaller crown sizes significantly lowered procedural complications, including spasm, embolism, and slow flow, thus underscoring the importance of technique optimization in utilizing this device.75 Orbital atherectomy followed by balloon angioplasty compared to PTA alone in infrapopliteal disease demonstrated better procedural success (93.1% vs 82.4%; P=.27) with less bail-out stenting and greater freedom from target vessel revascularization (TVR) (93.3% vs 80.0%; P=.14).76 A similar study comparing orbital atherectomy followed by PTA with PTA alone in calcified femoropopliteal disease showed freedom from TLR or restenosis of 77.1% vs 11.5% at 6 months (P<.001), inferring that plaque debulking with orbital atherectomy seems to increase the chance of attaining a desirable angioplasty result.77
Laser technology has also been utilized for the experimental ablation of atherosclerosis for decades.78 Although previous iterations of laser technology resulted in excessive thermal injury due to continuous power output, xenon chloride excimer laser delivers pulsed energy over a shorter period of time, allowing for ablation with less restenosis and thrombosis.79 The LACI trial, a prospective trial involving guidewire traversal and excimer laser angioplasty followed by PTA with optional stenting in patients who were poor candidates for bypass surgery, reported procedural success in 86% of treated lesions, with limb salvage of 92% at 6 months.80 The 8 Fr Turbo-Booster laser guide catheter (Spectranetics Corporation) allows for directional ablation along multiple planes resulting in more targeted ablation (Figure 4).81 The CELLO study revealed patency rates of 59% and 54% at 6 months and 12 months, respectively, and total TLR of 23.1% at 1 year for femoropopliteal lesions.81 A retrospective review of patients with both lower limb claudication and CLI evaluating for hard clinical endpoints underscore the need for an appropriately selected patient when intervening with laser atherectomy, because negative outcomes are seen in patients with comorbidities such as renal failure and diabetes mellitus, even when initial success rates are high.82
Although atherectomy is a promising interventional modality, a Cochrane Collaboration meta-analysis including a total of 220 patients has established that there is no clear evidence to currently support atherectomy over PTA for the outcome of primary patency over any interval of time.83 Additionally, the quality of evidence on atherectomy is poor, because randomized controlled trials that have been performed thus far are nonblinded, improperly powered, and have limited data on adverse events, making the true outcomes of this modality imprecise.83
Other technologies, such as cryoplasty, may play an adjunctive role in PVI. In a prospective, randomized, multicenter clinical trial of 74 diabetic patients with 90 SFA lesions undergoing BMS placement followed by postdilation using cryoplasty balloon vs conventional PTA, binary restenosis was significantly lower in the cryoplasty group (29.3% vs 55.8%; P=.01).84 A meta-analysis of 1,090 patients demonstrated that brachytherapy, which involves the application of radiation directly to the site of vessel narrowing after treatment, may be a useful adjunct for the purpose of maintaining patency and preventing restenosis.85
Economic Considerations
A physician study group on behalf of The Society for Cardiovascular Angiography and Interventions (SCAI) systematically reviewed the economics of the various endovascular modalities in PAD by using a Markov model to estimate the 24-month budget impact of index procedures and reinterventions in the United States.86 Index procedures were PTA, DEB, BMS, and DES while atherectomy was only considered as a technique for reintervention given the interest in noncomplex lesions for the analysis. Given the lack of generalizable evidence, there was no differentiation among the different atherectomy catheters.
A number of assumptions about the model were made for the purpose of the analysis. The choice of therapy in reintervention was based on the opinion of the authors, only one reintervention was allowed during the 24-month period, only one device was assumed to be used during an intervention, an assumption was made that stent-based or DCB procedures would implicate the use of an uncoated balloon for predilation, and amputations or deaths were not accounted for. Cost assumptions were also made.
The Medicare reimbursement rate for revascularization with DEB was assumed to be similar to PTA because DEB is not yet approved in the United States, and the reimbursement rate for revascularization with a DES was assumed to be similar to BMS because no separate reimbursement exists as of yet for these devices. Reimbursement rates were determined by 2013 national fiscal data for PVI. Also, a mix-adjusted average of Medicare severity diagnosis related groups (MS-DRGs) was used, with 50% of the procedures assumed to be performed in the inpatient setting. A total of 13 studies with 2,406 patients were included in this analysis. The average cost per patient over 24 months, including the cost of the index procedure and the possible cost of reintervention was lowest for DEB ($10,214) followed by DES ($12,904), PTA ($13,114) and BMS ($13,802). In their analysis, which also looked at clinical effectiveness, DEB and DES compared favorably to PTA and BMS from the perspective of 24-month TLR, with number-needed-to-treat in the 4 to 7 range per TLR avoided. On the basis of these data, drug-eluting strategies and particularly DEBs offer the lowest budget impact and highest economic value when concurrently accounting for clinic effectiveness. However, due to the assumptions made for the analysis, the results should be interpreted with caution.
Limitations of Current Data
While developments in the field of endovascular intervention have exciting implications, the heterogeneity of the available literature makes drawing definitive conclusions difficult. Many of the studies have been limited by design, including varying study size, study population, study location, type of imaging technique used, site of intervention, as well as the duration and intensity of antithrombotic use, all lending to a lack of gold-standard evidence. Operator and author interpretation of patient cases and preferences for treatment also produce bias that ultimately affects outcome data. Further, the use of disparate endpoints including patency, limb salvage, TLR, TVR, and freedom from reintervention makes the direct comparison of modalities problematic. Even with many of these limitations accounted for, it remains to be seen whether enhanced outcomes beyond 12 to 24 months without excess adverse events can be accomplished. While higher-quality studies are required to establish the genuine efficacy of these evolving modalities, improvements in device quality and technical expertise will be especially beneficial.
Conclusion
The current landscape for PVI includes various technologies that may improve clinical outcomes in PAD. The use of DEB increases patency and TLR outcomes in both femoropopliteal and infrapopliteal PAD. Stenting has a rising role in both bail-out and primary interventions and is particularly promising with the development of DES. The proposed advantages of atherectomy have not yet reliably translated into improved clinical outcomes, although the effectiveness of this approach will increase with technique optimization, refinement of the technology, and further study. Although the guidelines currently support PVI, particularly PTA with bail-out stenting conclusively in patients in a low TASC category who are otherwise not surgical candidates, further study of these emerging technologies in well-designed and highly powered trials are needed to determine the ideal treatment strategies for the management of patients with PAD.
Editor’s note: Disclosure: The authors have completed and returned the ICMJE Form for Disclosure of Potential Conflicts of Interest. The authors report no disclosures related to the content herein.
Manuscript received March 4, 2015; provisional acceptance given June 15, 2015; manuscript accepted July 9, 2015.
Address for correspondence: Michael S. Lee, MD, 100 Medical Plaza, Suite 630, Los Angeles, CA 90095, United States. E-mail: mslee@mednet.ucla.edu.
References
- Fowkes FG, Rudan D, Rudan I, et al. Comparison of global estimates of prevalence and risk factors for peripheral artery disease in 2000 and 2010: a systematic review and analysis. Lancet. 2013;382(9901):1329-1340.
- Norgren L, Hiatt WR, Dormandy JA, Nehler MR, Harris KA, Fowkes FG; TASC II Working Group. Inter-Society Consensus for the Management of Peripheral Arterial Disease (TASC II). J Vasc Surg. 2007;45 Suppl S:S5-S67
- Hirsch AT, Haskal ZJ, Hertzer NR, et al. ACC/AHA 2005 guidelines for the management of patients with peripheral arterial disease (lower extremity, renal, mesenteric, and abdominal aortic): executive summary a collaborative report from the American Association for Vascular Surgery/Society for Vascular Surgery, Society for Cardiovascular Angiography and Interventions, Society for Vascular Medicine and Biology, Society of Interventional Radiology, and the ACC/AHA Task Force on Practice Guidelines (Writing Committee to Develop Guidelines for the Management of Patients With Peripheral Arterial Disease) endorsed by the American Association of Cardiovascular and Pulmonary Rehabilitation; National Heart, Lung, and Blood Institute; Society for Vascular Nursing; TransAtlantic Inter-Society Consensus; and Vascular Disease Foundation. J Am Coll Cardiol. 2006;47(6):1239-1312.
- Anderson JL, Halperin JL, Albert NM, et al. Management of patients with peripheral artery disease (compilation of 2005 and 2011 ACCF/AHA guideline recommendations): a report of the American College of Cardiology Foundation/American Heart Association Task Force on Practice Guidelines. Circulation. 2013;127(13):1425-1443.
- Laird JR. Limitations of percutaneous transluminal angioplasty and stenting for the treatment of disease of the superficial femoral and popliteal arteries. J Endovasc Ther. 2006;13(Suppl II):II30-II40.
- Ansel GM, Lumsden AB. Evolving modalities for femoropopliteal interventions. J Endovasc Ther. 2009;16(Suppl II):II82-II97.
- Lo RC, Darling J, Bensley RP, et al. Outcomes following infrapopliteal angioplasty for critical limb ischemia. J Vasc Surg. 2013;57(6):1455-1463.
- Baumann F, Willenberg T, Do DD, Keo HH, Baumgartner I, Diehm N. Endovascular revascularization of below-the-knee arteries: prospective short-term angiographic and clinical follow-up. J Vasc Interv Radiol. 2011;22(12):1665-1673.
- Dormandy JA, Rutherford RB. Management of peripheral arterial disease (PAD). J Vasc Surg. 2000;31:S1-S296.
- Johnston KW. Femoral and popliteal arteries: reanalysis of results of balloon angioplasty. Radiology. 1992:183(3):767-771.
- Minar E, Pokrajac B, Maca T, et al. Endovascular brachytherapy for prophylaxis of restenosis after femoropopliteal angioplasty: results of a prospective randomized study. Circulation. 2000;102(22):2694-2699.
- Schmidt A, Ulrich M, Winkler B, et al. Angiographic patency and clinical outcome after balloon-angioplasty for extensive infrapopliteal arterial disease. Catheter Cardiovasc Interv. 2010;76(7):1047-1054.
- Conte MS, Geraghty PJ, Bradbury AW, et al. Suggested objective performance goals and clinical trial design for evaluating catheter-based treatment of critical limb ischemia. J Vasc Surg. 2009;50(6):1462-1473.
- Iida O, Soga Y, Kawasaki D, et al. Angiographic restenosis and its clinical impact after infrapopliteal angioplasty. Eur J Vasc Endovasc Surg. 2012;44(4):425-431.
- Bradbury AW, Adam DJ, Bell J, et al.; BASIL Trial Participants. Bypass versus Angioplasty in Severe Ischaemia of the Leg (BASIL) trial: A survival prediction model to facilitate clinical decision making. J Vasc Surg. 2010;51(5 Suppl):52S-68S.
- Latib A, Colombo A, Castriota F, et al. A randomized multicenter study comparing a paclitaxel drug-eluting balloon with a paclitaxel-eluting stent in small coronary vessels: the BELLO (Balloon Elution and Late Loss Optimization) study. J Am Coll Cardiol. 2012;60(24):2473-2480.
- Micari A, Cioppa A, Vadalà G, et al. Clinical evaluation of a paclitaxel-eluting balloon for treatment of femoropopliteal arterial disease: 12-month results from a multicenter Italian registry. JACC Cardiovasc Interv. 2012 Mar;5(3):331-338.
- Tepe G, Zeller T, Albrecht T, et al. Local delivery of paclitaxel to inhibit restenosis during angioplasty of the leg. N Engl J Med. 2008;358(7):689–699.
- Scheinert D, Duda S, Zeller T, et al. The LEVANT I (Lutonix paclitaxel-coated balloon for the prevention of femoropopliteal restenosis) trial for femoropopliteal revascularization: first-in-human randomized trial of low-dose drug-coated balloon versus uncoated balloon angioplasty. JACC Cardiovasc Interv. 2014;7(1):10–19.
- Tepe G, Schnorr B, Albrecht T, et al. Angioplasty of Femoral-Popliteal Arteries With Drug-Coated Balloons: 5-Year Follow-Up of the THUNDER Trial. JACC Cardiovasc Interv. 2015;8(1 Pt A):102-108.
- Schmidt A, Piorkowski M, Werner M, et al. First experience with drug-eluting balloons in infrapopliteal arteries restenosis rate and clinical outcome. J Am Coll Cardiol. 2011;58(11):1105–1109.
- Liistro F, Porto I, Angioli P, et al. Drug-Eluting Balloon in Peripheral Intervention for Below the Knee Angioplasty Evaluation (DEBATE-BTK): a randomized trial in diabetic patients with critical limb ischemia. Circulation. 2013;128(6):615–621.
- Zeller T, Baumgartner I, Scheinert D, et al.; IN.PACT DEEP Trial Investigators. Drug-eluting balloon versus standard balloon angioplasty for infrapopliteal arterial revascularization in critical limb ischemia: 12-month results from the IN.PACT DEEP randomized trial. J Am Coll Cardiol. 2014;64(15):1568-1576.
- Laird JR, Armstrong EJ. Drug-coated balloons for infrapopliteal disease: digging deep to understand the impact of a negative trial. J Am Coll Cardiol. 2014;64(15):1577-1579.
- Rosenfield K, Jaff MR, White CJ, et al.; LEVANT 2 Investigators. Trial of a Paclitaxel-Coated Balloon for Femoropopliteal Artery Disease. N Engl J Med. 2015;373(2):145-153.
- Yang X, Lu X, Ye K, Li X, Qin J, Jiang M. Systematic review and meta-analysis of balloon angioplasty versus primary stenting in the infrapopliteal disease. Vasc Endovascular Surg. 2014;48(1):18-26.
- Hiro R, Leung CY, de Guzman S, Gordon IL, Conroy RM, Tobis JM. A comparison of balloon angioplasty and self-expandable stent treatment of occluded superficial femoral arteries. Circulation. 1995;92(8):1–56.
- Schillinger M, Sabeti S, Loewe C, et al. Balloon angioplasty versus implantation of nitinol stents in the superficial femoral artery. N Engl J Med. 2006;354(18):1879-1888.
- Schillinger M, Sabeti S, Dick P, et al. Sustained benefit at 2 years of primary femoropopliteal stenting compared with balloon angioplasty with optional stenting. Circulation. 2007;115(21):2745-2749.
- Soga Y, Iida O, Hirano K, Yokoi H, Nanto S, Nobuyoshi M. Mid-term clinical outcome and predictors of vessel patency after femoropopliteal stenting with self-expandable nitinol stent. J Vasc Surg. 2010;52(3):608–615.
- Gray WA, Feiring A, Cioppi M, et al.; STROLL Study Investigators. S.M.A.R.T. Self-Expanding Nitinol Stent for the Treatment of Atherosclerotic Lesions in the Superficial Femoral Artery (STROLL): 1-Year Outcomes. J Vasc Interv Radiol. 2015;26(1):21-28.
- Biondi-Zoccai GG, Sangiorgi G, Lotrionte M, et al. Infragenicular stent implantation for below-the-knee atherosclerotic disease: clinical evidence from an international collaborative meta-analysis on 640 patients. J Endovasc Ther. 2009;16(3):251-260.
- Link J, Müller-Hülsbeck S, Brossmann J, Steffens JC, Heller M. Perivascular inflammatory reaction after percutaneous placement of covered stents. Cardiovasc Intervent Radiol. 1996;19(5):345-347.
- Maynar M, Reyes R, Ferral H, et al. Cragg Endopro System I: early experience. I. Femoral arteries. J Vasc Interv Radiol. 1997;8(2):203-207.
- Saxon RR, Coffman JM, Gooding JM, Ponec DJ. Long-term patency and clinical outcome of the Viabahn stent-graft for femoropopliteal artery obstructions. J Vasc Interv Radiol. 2007;18(11):1341-1349.
- Alimi YS, Hakam Z, Hartung O, et al. Efficacy of Viabahn in the treatment of severe superficial femoral artery lesions: which factors influence long-term patency? Eur J Vasc Endovasc Surg. 2008;35(3):346-352.
- Lammer J, Dake MD, Bleyn J, et al. Peripheral arterial obstruction: prospective study of treatment with a transluminally placed self-expanding stent-graft. International Trial Study Group. Radiology. 2000;217(1):95-104.
- Deutschmann HA, Schedlbauer P, Bérczi V, Portugaller H, Tauss J, Hausegger KA. Placement of Hemobahn stent-grafts in femoropopliteal arteries: early experience and midterm results in 18 patients. J Vasc Interv Radiol. 2001;12(8):943-950.
- Railo M, Roth WD, Edgren J, et al. Preliminary results with endoluminal femoropopliteal thrupass. Ann Chir Gynaecol. 2001;90(1):15-18.
- Kedora J, Hohmann S, Garrett W, Munschaur C, Theune B, Gable D. Randomized comparison of percutaneous Viabahn stent grafts vs prosthetic femoral-popliteal bypass in the treatment of superficial femoral arterial occlusive disease. J Vasc Surg. 2007;45(1):10-16.
- Saxon RR, Dake MD, Volgelzang RL, Katzen BT, Becker GJ. Randomized, multicenter study comparing expanded polytetrafluoroethylene-covered endoprosthesis placement with percutaneous transluminal angioplasty in the treatment of superficial femoral artery occlusive disease. J Vasc Interv Radiol. 2008;19(6):823-832.
- Geraghty PJ, Mewissen MW, Jaff MR, Ansel GM; VIBRANT Investigators. Three-year results of the VIBRANT trial of VIABAHN endoprosthesis versus bare nitinol stent implantation for complex superficial femoral artery occlusive disease. J Vasc Surg. 2013;58(2):386-395.
- Peeters P, Verbist J, Deloose K, Bosiers M. Results with heparin bonded polytetrafluoroethylene grafts for femorodistal bypasses. J Cardiovasc Surg (Torino). 2006;47(4):407-413.
- Bosiers M, Deloose K, Verbist J, et al. Heparin-bonded expanded polytetrafluoroethylene vascular graft for femoropopliteal and femorocrural bypass grafting: 1-year results. J Vasc Surg. 2006;43(2):318-319.
- Lammer J, Zeller T, Hausegger KA, et al. Sustained benefit at 2 years for covered stents versus bare-metal stents in long SFA lesions: the VIASTAR trial. Cardiovasc Intervent Radiol. 2015;38(1):25-32.
- Rosenthal D, Martin JD, Smeets L, et al. Remote superficial femoral artery endarterectomy and distal aSpire stenting: results of a multinational study at three-year follow-up. J Cardiovasc Surg (Torino). 2006;47(4):385-391.
- Lenti M, Cieri E, De Rango P, et al. Endovascular treatment of long lesions of the superficial femoral artery: results from a multicenter registry of a spiral, covered polytetrafluoroethylene stent. J Vasc Surg. 2007;45(1):32-39.
- Duda SH, Bosiers M, Lammer J, et al. Sirolimus-eluting versus bare nitinol stent for obstructive superficial femoral artery disease: the SIROCCO II trial. J Vasc Interv Radiol. 2005;16(3):331-338.
- Duda SH, Bosiers M, Lammer J, et al. Drug-eluting and bare nitinol stents for the treatment of atherosclerotic lesions in the superficial femoral artery: long-term results from the SIROCCO trial. J Endovasc Ther. 2006;13(6):701-710.
- Dake M. The Zilver PTX randomized trial of treating femoropopliteal artery disease: 5-year results. Presented at: Vascular Interventional Advances (VIVA); November 4-7, 2014; Las Vegas, NV.
- Walker JP, Owens CD. Current Status of Drug-Eluting Stents and Drug-Eluting Balloons for the Superficial Femoral Artery. Curr Surg Rep. 2013;1(2):90-97.
- Dake MD, Ansel GM, Jaff MR, et al; Zilver PTX Investigators. Sustained safety and effectiveness of paclitaxel-eluting stents for femoropopliteal lesions: 2-year follow-up from the Zilver PTX randomized and single-arm clinical studies. J Am Coll Cardiol. 2013;61(24):2417-2427.
- Lammer J, Bosiers M, Zeller T, et al. First clinical trial of nitinol self-expanding everolimus-eluting stent implantation for peripheral arterial occlusive disease. J Vasc Surg. 2011;54(2):394-401.
- Rastan A, Noory E, Zeller T. Drug-eluting stents for treatment of focal infrapopliteal lesions. Vasa. 2012;41(2):90-05.
- Scheinert D, Katsanos K, Zeller T, et al; ACHILLES Investigators. A prospective randomized multicenter comparison of balloon angioplasty and infrapopliteal stenting with the sirolimus-eluting stent in patients with ischemic peripheral arterial disease: 1-year results from the ACHILLES trial. J Am Coll Cardiol. 2012;60(22):2290-2295.
- Rastan A, Tepe G, Krankenberg H, et al. Sirolimus-eluting stents vs. bare-metal stents for treatment of focal lesions in infrapopliteal arteries: a double-blind, multi-centre, randomized clinical trial. Eur Heart J. 2011;32(18):2274-2281.
- Bosiers M, Scheinert D, Peeters P, et al. Randomized comparison of everolimus-eluting versus bare-metal stents in patients with critical limb ischemia and infrapopliteal arterial occlusive disease. J Vasc Surg. 2012;55(2):390-398.
- Karnabatidis D, Spiliopoulos S, Diamantopoulos A, et al. Primary everolimus-eluting stenting versus balloon angioplasty with bailout bare metal stenting of long infrapopliteal lesions for treatment of critical limb ischemia. J Endovasc Ther. 2011;18(1):1-12.
- Zeller T, Rastan A, Macharzina R, et al. Drug-coated balloons vs. drug-eluting stents for treatment of long femoropopliteal lesions. J Endovasc Ther. 2014;21(3):359-368.
- Laird JR. Endovascular treatment of common femoral artery disease viable alternative to surgery or just another short-term fix. J Am Coll Cardiol. 2011;58(8):799-800.
- Henry M, Klonaris C, Amor M, Henry I, Tzvetanov K. State of the art: which stent for which lesion in peripheral interventions? Tex Heart Inst J. 2000;27(2):119-126.
- Laird JR, Yeo KK. The treatment of femoropopliteal in-stent restenosis: back to the future. J Am Coll Cardiol. 2012;59(1):24-25.
- Höfling B, Pölnitz AV, Backa D, et al. Percutaneous removal of atheromatous plaques in peripheral arteries. Lancet. 1988;1(8582):384-386.
- Zeller T, Rastan A, Sixt S, et al. Long-term results after directional atherectomy of femoro-popliteal lesions. J Am Coll Cardiol. 2006;48(8):1573-1578.
- Biskup NI, Ihnat DM, Leon LR, Gruessner AC, Mills JL. Infrainguinal atherectomy: a retrospective review of a single-center experience. Ann Vasc Surg. 2008;22(6):776-782.
- Sarac TP, Altinel O, Bannazadeh M, Kashyap V, Lyden S, Clair D. Midterm outcome predictors for lower extremity atherectomy procedures. J Vasc Surg. 2008;48(4):885-890.
- Werner-Gibbings K, Dubenec S. Short-term outcomes of excisional atherectomy in lower limb arterial disease. ANZ J Surg. 2014 Nov 3. [Epub ahead of print]
- Spencer B, Yeung AC. Rotational atherectomy: concepts and practice. In: Interventional Cardiology. New York: McGraw-Hill; 2007:333-347.
- Reifart N, Vandormael M, Krajcar M, et al. Randomized comparison of angioplasty of complex coronary lesions at a single center. Excimer Laser, Rotational Atherectomy, and Balloon Angioplasty Comparison (ERBAC) Study. Circulation. 1997;96(1):91-98.
- Tran T, Brown M, Lasala J. An evidence-based approach to the use of rotational an directional coronary atherectomy in the era of drug-eluting stents: when does it make sense? Catheter Cardiovasc Interv. 2008;72(5):650-662.
- Zeller T, Krankenberg H, Rastan A, et al. Percutaneous rotational and aspiration atherectomy in infrainguinal peripheral arterial occlusive disease: a multicenter pilot study. J Endovasc Ther. 2007;14(3):357-364.
- Zeller T, Krankenberg H, Steinkamp H, et al. One-year outcome of percutaneous rotational atherectomy with aspiration in infrainguinal peripheral arterial occlusive disease: the multicenter pathway PVD trial. J Endovasc Ther. 2009;16(6):653-662.
- Shrikhande GV, Khan SZ, Hussain HG, Dayal R, McKinsey JF, Morrissey N. Lesion types and device characteristics that predict distal embolization during percutaneous lower extremity interventions. J Vasc Surg. 2011;53(2):347-352.
- Heuser RR. Treatment of lower extremity vascular disease: the Diamondback 360 degrees Orbital Atherectomy System. Expert Rev Med Devices. 2008;5(3):279–286.
- Das T, Mustapha J, Indes J, et al. Technique optimization of orbital atherectomy in calcified peripheral lesions of the lower extremities: the CONFIRM series, a prospective multicenter registry. Catheter Cardiovasc Interv. 2014;83(1):115-122.
- Shammas NW, Lam R, Mustapha J, et al. Comparison of orbital atherectomy plus balloon angioplasty vs. balloon angioplasty alone in patients with critical limb ischemia: results of the CALCIUM 360 randomized pilot trial. J Endovasc Ther. 2012;19(4):480-488.
- Dattilo R, Himmelstein SI, Cuff RF. The COMPLIANCE 360° Trial: a randomized, prospective, multicenter, pilot study comparing acute and long-term results of orbital atherectomy to balloon angioplasty for calcified femoropopliteal disease. J Invasive Cardiol. 2014;26(8):355-360.
- Choy DS. History of lasers in medicine. Thorac Cardiovasc Surg. 1988;36 Suppl 2:114-117.
- Biamino G. The excimer laser: science fiction fantasy or practical tool? J Endovasc Ther. 2004;11 Supl 2:II207-II222.
- Laird JR, Zeller T, Gray BH, et al.; LACI Investigators. Limb salvage following laser-assisted angioplasty for critical limb ischemia: results of the LACI multicenter trial. J Endovasc Ther. 2006;13(1):1-11.
- Dave RM, Patlola R, Kollmeyer K, et al.; CELLO Investigators. Excimer laser recanalization of femoropopliteal lesions and 1-year patency: results of the CELLO registry. J Endovasc Ther. 2009;16(6):665-675.
- Stoner MC, deFreitas DJ, Phade SV, Parker FM, Bogey WM, Powell S. Mid-term results with laser atherectomy in the treatment of infrainguinal occlusive disease. J Vasc Surg. 2007 Aug;46(2):289-295.
- Ambler GK, Radwan R, Hayes PD, Twine CP. Atherectomy for peripheral arterial disease. Cochrane Database Syst Rev. 2014;3:CD006680.
- Banerjee S, Das TS, Abu-Fadel MS, et al. Pilot trial of cryoplasty or conventional balloon post-dilation of nitinol stents for revascularization of peripheral arterial segments: the COBRA trial. J Am Coll Cardiol. 2012;60(15):1352-1359.
- Andras A, Hansrani M, Stewart M, Stansby G. Intravascular brachytherapy for peripheral vascular disease. Cochrane Database Syst Rev. 2014;1:CD003504.
- Pietzsch JB, Geisler BP, Garner AM, Zeller T, Jaff MR. Economic analysis of endovascular interventions for femoropopliteal arterial disease: a systematic review and budget impact model for the United States and Germany. Catheter Cardiovasc Interv. 2014;84(4):546-554.