Advances and Novel Approaches in Mapping and Ablation of Ventricular Tachycardia
© 2023 HMP Global. All Rights Reserved.
Any views and opinions expressed are those of the author(s) and/or participants and do not necessarily reflect the views, policy, or position of EP Lab Digest or HMP Global, their employees, and affiliates.
EP LAB DIGEST. 2023;23(5):20-24.
The increasing burden of ventricular tachycardia (VT) is a by-product of one of the greatest success stories in cardiology. Our improved ability to manage patients with acute coronary syndrome has resulted in patients surviving their initial presentation with varying degrees of cardiac damage and an increased risk of scar-related VT. The use of implantable cardioverter-defibrillators (ICDs) in this cohort with severe left ventricular systolic dysfunction has been shown to increase survival as approximately 15% of patients with primary prevention ICDs receive appropriate ICD shocks resulting in a 3-fold increased mortality.1-4 With this increasing burden of appropriate ICD therapies, greater emphasis has been placed on developing advanced tools and methodologies to manage VT. In this review, we aim to highlight some of the recent advances in invasive mapping and ablation of VT, and how they are likely to impact future practice.
Novel Ablation Tools
Radiofrequency (RF) catheter ablation is an established treatment for VT. Regardless of the arrhythmia and targeted tissue, lesion durability and arrhythmia recurrence remain prominent issues.5,6 The technique of utilizing high-power, short-duration (HPSD) ablation has emerged, in which energies ≥50W are delivered for durations of 15 seconds or less. The resulting lesions are comparable in volume to traditional irrigated RF, though generally wider and shallower.7 Similar to these HPSD settings, temperature-controlled irrigated RF can operate with a power limit of 50W; however, the power will modulate to maintain a predetermined temperature set point, measured by thermocouples. Temperature-controlled irrigated RF has been developed to facilitate ‘tailored’ power delivery based on the catheter tip-surface tissue temperature interface and has been utilized in several ablation catheters, including the DiamondTemp (Medtronic), QDot Micro (Biosense Webster, Inc, a Johnson & Johnson company) and Sphere-9 (Affera, a Medtronic company) in RF mode. The DiamondTemp catheter has 6 externalized thermocouples and chemical vapor deposit industrial diamonds embedded within the catheter tip that act as a thermal shunt and relays temperature 200-400 times faster than standard platinum-iridium. The QDot catheter uses 6 thermocouples embedded within the catheter tip, enabling temperature-controlled RF ablation due to improved temperature feedback.8 The Sphere-9 cardiac ablation and mapping catheter is a novel lattice-tip pulsed field ablation (PFA) catheter with 9 surface thermocouples, providing temperature feedback for additional temperature-controlled RF ablation. Although these catheters have been studied with success within the atrium, data for VT ablation in humans is limited to the DiamondTemp ablation catheter.9
Our group published the first reported series of DiamondTemp ablation in structural heart VT, with temperature-controlled RF delivered (max 50W) for 45 seconds per lesion.9 An 88% reduction in total VT episodes and 77% reduction in ICD therapies at 6-month follow-up were reported. More recently, optimization studies of the ablation protocol utilizing a time from target-temperature approach, with ablation limited to 20 seconds once the set-target temperature of 60 °C was reached, resulted in a 58% reduction in total RF time with comparable efficacy data (unpublished data).
PFA is an emerging technology that provides an alternative energy source for cardiac ablation. PFA ablates tissue nonthermally by creating micropores within the cell membrane, in a tissue-selective process, which reduces the risk of collateral damage to nearby structures such as the esophagus and nerve tissue.10,11 A number of trials have investigated the use of PFA in atrial ablation, with promising results.10,12,13 However, the use of PFA in the ventricle has been limited. The ability to create effective lesions has been demonstrated in animal infarct models, but further translation to human trials is still required.11
Functional Substrate Identification
Successful VT ablation relies on the identification of key components of the VT circuit. Entrainment and activation mapping are the gold standard for identifying the critical isthmus of the reentrant VT circuit, but are limited by VT inducibility and hemodynamic instability.14 Voltage mapping can identify border zone and dense scar, along with late potentials (LPs) and local abnormal ventricular activity (LAVA).15 However, this identifies areas that may or may not be relevant to the VT circuit, consequently leading to a larger ablation target area.
Functional substrate mapping aims to identify critical isthmus components without inducing VT by highlighting substrate utilizing an extrastimulus (ES).14 The short-coupled ES identifies areas with critical conduction delay; these regions are more susceptible to unidirectional block and reentry, and are more likely to play a key role in the clinical VT circuit (Figure 1). Functional substrate mapping techniques include decrement evoked potential (DEEP) mapping, isochronal late activation mapping (ILAM), and paced electrogram feature analysis (PEFA).16-18 These techniques provide an exciting alternative to “traditional” VT mapping.
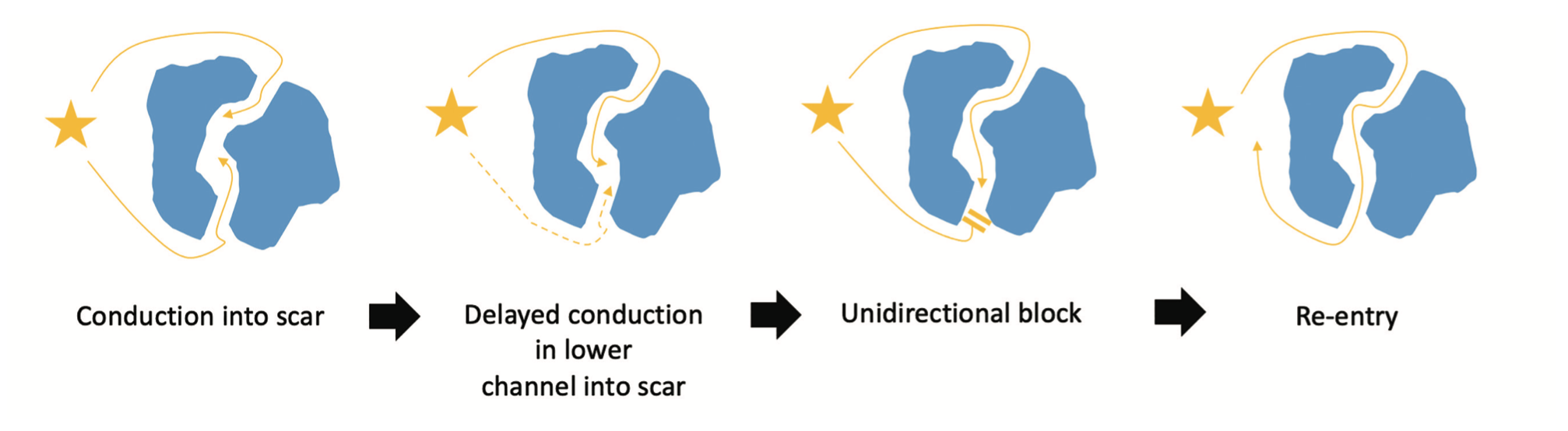
DEEP mapping involves the identification of local LPs that delay when an ES (S2) is delivered through a catheter placed in the right ventricular apex (RVA), after a short drive train (S1). A delay of greater than 10 milliseconds has been used as the cut-off for significant decrement (Figure 2), which defines a “DEEP.”19,20 Porta-Sánchez et al delivered an ES at 20 milliseconds above the ventricular effective refractory period (VERP+20 milliseconds), with a preceding drive train at 600 milliseconds.16 DEEP-guided ablation was shown to have an improved specificity for identifying the VT critical isthmus than LP mapping, with a significant reduction in VT burden at 6-month follow-up.20 Shariat et al also examined intracardiac electrograms (EGM) during RVA pacing with an S2 at VERP+50/100/150 milliseconds after a 600-millisecond drive train.18 Unlike Porta-Sánchez et al, they examined the EGM duration, measured from the S2 pacing artifact to the last deflection, looking for an increase in both the latency (LT) and EGM duration (ED). Using a cohort of 19 patients (5 controls), they were able to define the upper limit of normal for LT and ED as 45 and 120 milliseconds, respectively. Using the LT and ED data, the authors constructed PEFA maps to highlight functional substrate. In 10 patients, RFA was guided by PEFA maps generated with an S2 at VERP+50 milliseconds and ED of >120 milliseconds. All patients were free from VT at the end of the procedure, with 9 patients free from recurrence during follow-up (345±90 days).
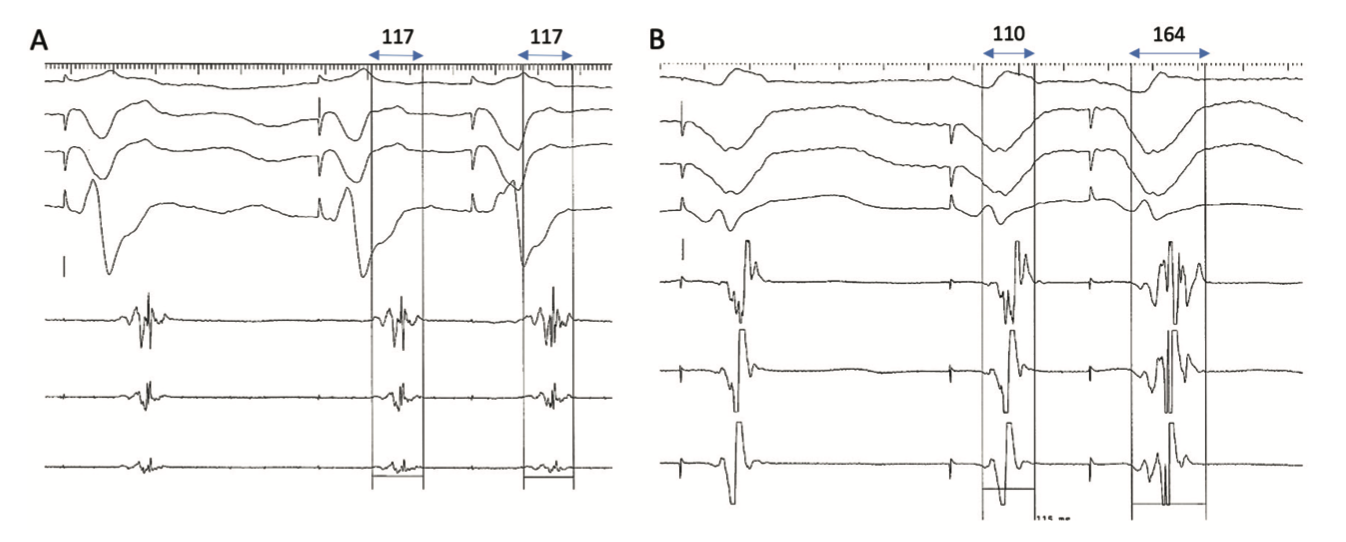
ILAM identifies regions of slow conduction (deceleration zones [DZ]) during sinus rhythm. Irie et al divided their electroanatomical maps (EAM) into 8 isochrones, each accounting for 12.5% of ventricular activation.21 A strong correlation was observed between critical sites for reentry and DZ, which were defined as regions of isochronal crowding (>2 isochrones within a 1-cm radius). A prospective study of 120 patients by Aziz et al assessed the outcomes of ILAM-guided RFA targeting DZ (>3 isochrones within a 1-cm radius), and demonstrated a 70% freedom from VT recurrence at 12±10 month follow-up.17
Noninvasive mapping techniques have also been used to highlight conduction channels within myocardial scar, which can aid the planning process and guide noninvasive ablation (eg, stereotactic ablative radiotherapy) in VT. Automated detection of arrhythmic substrate (ADAS 3D) software has been used to process late gadolinium enhancement cardiac magnetic resonance imaging to highlight conduction corridors within regions of dense scar.22 Ongoing studies utilizing the novel View Into Ventricular Onset (VIVO) mapping system (Catheter Precision) will determine whether this noninvasive system can accurately predict the VT exit site in scar-dependent reentrant VT.
Data Mining Using Open-Source Platforms
During VT ablation procedures, vast arrays of data are collected using EAM systems. Using contemporary EAM systems, it is not uncommon for more than 2500-5000 EGM points to be recorded, including both EGM and positional data. Mapping can be performed during sinus rhythm, paced rhythm, and VT, providing numerous datasets quantifying ventricular EP.2,16-18 Storage, retrieval, and analysis of these datasets represents a major barrier to research into pathophysiological mechanisms of VT for 2 main reasons. First, the data are typically stored in proprietary formats that are inaccessible for research. Second, these formats are usually space inefficient, with the data running to several gigabytes per patient.
Tools such as OpenEP and EnPlot (v3.4) are open-source platforms for EP data analysis designed to overcome these limitations.23 OpenEP provides data parsing modules for the main EAM systems, together with a suite of tools for analysis of EAM data (Figure 3A). A graphical interface for OpenEP, called EP Workbench, has recently been described to allow visualization and manipulation of EAM data offline from clinical EAM platforms (Figure 3B), and is undergoing beta testing.
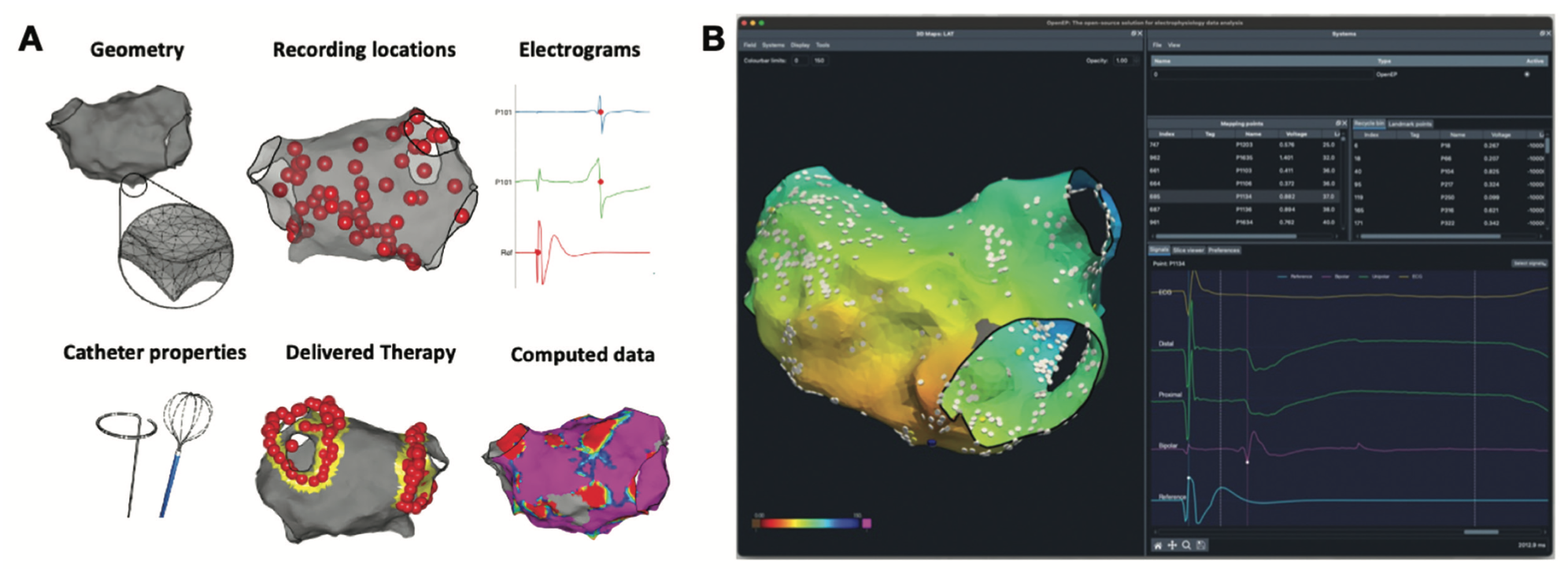
Relevant to VT research, OpenEP provides tools for assessment of both EGM voltage and conduction velocity. Tools are also provided to permit segmentation of EAM models to permit regional analysis. In a recent single-center study, OpenEP was used to assess conduction velocity in patients undergoing cardiac stereotactic body radiotherapy (SBRT) for refractory VT. In several preclinical animal studies, cardiac SBRT has been shown to influence cardiac conduction in the early post-therapy period by increasing cardiac conduction velocity through increased sodium channel and gap junction expression.24 By using the regional analysis and conduction velocity tools, Whitaker et al found that a single 25 Gy fraction resulted in reduced conduction velocity in the exposed myocardium at 1 year following cardiac SBRT.25 The results suggest that the effects of cardiac fibrosis may predominate over those of ion channel and gap junction expression at this time point, highlighting the need for further research in this area.
More recently, we have used EnPlot (v3.4) for bespoke offline analysis of omnipolar EGMs from the Advisor HD Grid Mapping Catheter, Sensor Enabled (Abbott) that were exported along with anatomic data. There is currently no mapping system that enables the automated creation of a DEEP map; however, using EnPlot, offline DEEP maps were generated (Figure 4). To create the DEEP map, last deflection minus first deflection timing maps to generate EGM duration were computed directly from the exported EGMs for the reference cycle length (CL) of 600 milliseconds (S1), labeled as S1CL and the S2, labeled as S2CL. DEEP maps, in this case with a decrement threshold of >10 milliseconds, highlighted by the purple area, were generated from the difference in EGM duration between the S2CL beat minus the S1CL drive train beat.
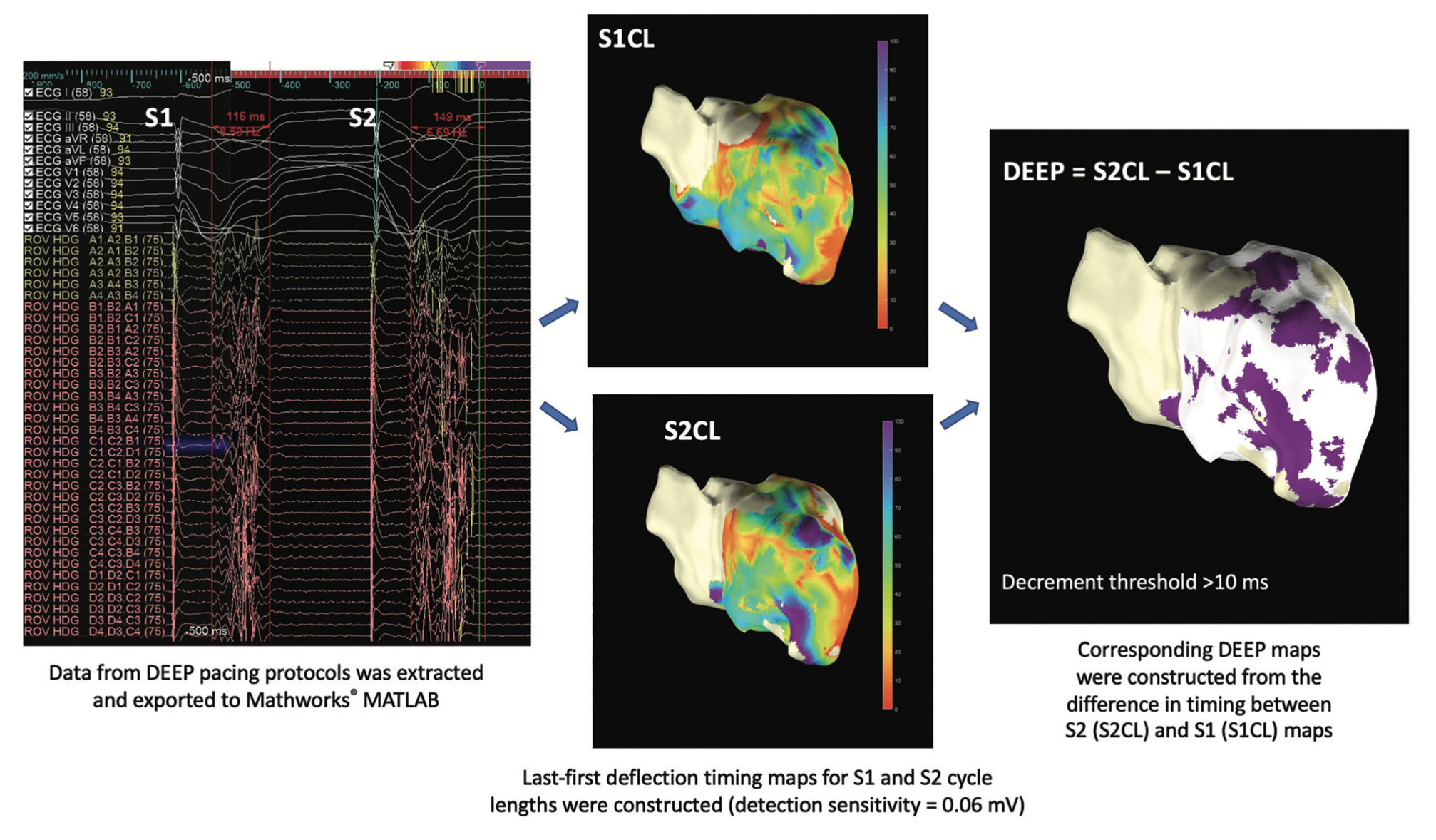
Another recent advance in therapy for VAs is the use of computational modelling to predict arrhythmia mechanisms and define therapeutic targets. In a recent study, Campos et al described the Virtual Induction and Treatment of Arrhythmias (VITA) method to identify ablation targets in scar-related VT, using preprocedural cardiac imaging to identify ischemic scar.26 Conversely, in the setting of atrial arrhythmias, EAM data encoded via OpenEP has been extensively studied for the calibration of atrial computational models. It is now possible to implement computational arrhythmia models directly via OpenEP/EP Workbench. Ongoing work will develop methods to run computational simulations on the UK national supercomputing resource (ARCHER2) directly from EP Workbench. This advance will lead to the intriguing possibility of incorporating computational modelling, based on EAM data, directly into clinical workflows for the management of VT.
Conclusion
The management of VT is a rapidly changing landscape with the introduction of new software and technologies. There are many recent developments in VT mapping and ablation, with many exciting areas for future research.
Funding sources: SEW is supported by the British Heart Foundation (FS/20/26/34952).
Disclosure: The authors have completed and returned the ICMJE Form for Disclosure of Potential Conflicts of Interest. Dr Dhanjal reports receiving research grants from Medtronic and Abbott.
References
1. Zeppenfeld K, Tfelt-Hansen J, de Riva M, et al. 2022 ESC guidelines for the management of patients with ventricular arrhythmias and the prevention of sudden cardiac death. Eur Heart J. 2022;43(40):3997-4126. doi:10.1093/eurheartj/ehac262
2. Proietti R, Dowd R, Gee LV, et al. Impact of a high-density grid catheter on long-term outcomes for structural heart disease ventricular tachycardia ablation. J Interv Card Electrophysiol. 2021;62(3):519-529. doi:10.1007/s10840-020-00918-4
3. Bella PD, Baratto F, Vergara P, et al. Does timing of ventricular tachycardia ablation affect prognosis in patients with an implantable cardioverter defibrillator? Results from the multicenter randomized PARTITA trial. Circulation. 2022;145(25):1829-1838. doi:10.1161/CIRCULATIONAHA.122.059598
4. Arenal Á, Ávila P, Jiménez-Candil J, et al. Substrate ablation vs antiarrhythmic drug therapy for symptomatic ventricular tachycardia. J Am Coll Cardiol. 2022;79(15):1441-1453. doi:10.1016/j.jacc.2022.01.050
5. McLellan AJ, Ling LH, Ruggiero D, et al. Pulmonary vein isolation: the impact of pulmonary venous anatomy on long-term outcome of catheter ablation for paroxysmal atrial fibrillation. Heart Rhythm. 2014;11(4):549-556. doi:10.1016/j.hrthm.2013.12.025
6. Sapp JL, Wells GA, Parkash R, et al. Ventricular tachycardia ablation versus escalation of antiarrhythmic drugs. N Engl J Med. 2016;375(2):111-121. doi:10.1056/NEJMoa1513614
7. Bourier F, Duchateau J, Vlachos K, et al. High-power short-duration versus standard radiofrequency ablation: insights on lesion metrics. J Cardiovasc Electrophysiol. 2018;29(11):1570-1575. doi:10.1111/jce.13724
8. Wielandts JY, Almorad A, Hilfiker G, et al. Biosense Webster’s QDOT Micro™ radiofrequency ablation catheter. Future Cardiol. 2021;17(5):817-825. doi:10.2217/fca-2021-0002
9. Al-Sheikhli J, Patchett I, Lim VG, et al. Initial experience of temperature-controlled irrigated radiofrequency ablation for ischaemic cardiomyopathy ventricular tachycardia ablation. J Interv Card Electrophysiol. 2022 Feb 22. doi:10.1007/s10840-022-01158-4
10. Reddy VY, Koruth J, Jais P, et al. Ablation of atrial fibrillation with pulsed electric fields. JACC Clin Electrophysiol. 2018;4(8):987-995. doi:10.1016/j.jacep.2018.04.005
11. Kawamura I, Reddy VY, Wang BJ, et al. Pulsed field ablation of the porcine ventricle using a focal lattice-tip catheter. Circ Arrhythm Electrophysiol. 2022;15(9):e011120. doi:10.1161/CIRCEP.122.011120
12. Reddy VY, Neuzil P, Koruth JS, et al. Pulsed field ablation for pulmonary vein isolation in atrial fibrillation. J Am Coll Cardiol. 2019;74(3):315-326. doi:10.1016/j.jacc.2019.04.021
13. Reddy VY, Anter E, Rackauskas G, et al. Lattice-tip focal ablation catheter that toggles between radiofrequency and pulsed field energy to treat atrial fibrillation. Circ Arrhythm Electrophysiol. 2020;13(6):e008718. doi:10.1161/CIRCEP.120.008718
14. Papageorgiou N, Srinivasan NT. Dynamic high-density functional substrate mapping improves outcomes in ischaemic ventricular tachycardia ablation: sense protocol functional substrate mapping and other functional mapping techniques. Arrhythm Electrophysiol Rev. 2021;10(1):38-44. doi:10.15420/aer.2020.28
15. Jaïs P, Maury P, Khairy P, et al. Elimination of local abnormal ventricular activities: a new end point for substrate modification in patients with scar-related ventricular tachycardia. Circulation. 2012;125(18):2184-2196. doi:10.1161/CIRCULATIONAHA.111.043216
16. Porta-Sánchez A, Jackson N, Lukac P, et al. Multicenter study of ischemic ventricular tachycardia ablation with decrement-evoked potential (DEEP) mapping with extra stimulus. JACC Clin Electrophysiol. 2018;4(3):307-315. doi:10.1016/j.jacep.2017.12.005
17. Aziz Z, Shatz D, Raiman M, et al. Targeted ablation of ventricular tachycardia guided by wavefront discontinuities during sinus rhythm: a new functional substrate mapping strategy. Circulation. 2019;140(17):1383-1397. doi:10.1161/CIRCULATIONAHA.119.042423
18. Shariat MH, Gupta D, Gul EE, et al. Ventricular substrate identification using close-coupled paced electrogram feature analysis. Europace. 2019;21(3):492-501. doi:10.1093/europace/euy265
19. Jackson N, Gizurarson S, Viswanathan K, et al. Decrement evoked potential mapping: basis of a mechanistic strategy for ventricular tachycardia ablation. Circ Arrhythm Electrophysiol. 2015;8(6):1433-442. doi:10.1161/CIRCEP.115.003083
20. Srinivasan NT, Garcia J, Schilling RJ, et al. Multicenter study of dynamic high-density functional substrate mapping improves identification of substrate targets for ischemic ventricular tachycardia ablation. JACC Clin Electrophysiol. 2020;6(14):1783-1793. doi:10.1016/j.jacep.2020.06.037
21. Irie T, Yu R, Bradfield JS, et al. Relationship between sinus rhythm late activation zones and critical sites for scar-related ventricular tachycardia: systematic analysis of isochronal late activation mapping. Circ Arrhythm Electrophysiol. 2015;8(2):390-399. doi:10.1161/CIRCEP.114.002637
22. Bertagnolli L, Torri F, Paetsch I, et al. Cardiac magnetic resonance imaging for coregistration during ablation of ischemic ventricular tachycardia for identification of the critical isthmus. HeartRhythm Case Rep. 2018;4(2):70-72. doi:10.1016/j.hrcr.2017.11.008
23. Williams SE, Roney CH, Connolly A, et al. OpenEP: a cross-platform electroanatomic mapping data format and analysis platform for electrophysiology research. Front Physiol. 2021 Feb 26;12:646023. doi:10.3389/fphys.2021.646023
24. Zhang DM, Navara R, Yin T, et al. Cardiac radiotherapy induces electrical conduction reprogramming in the absence of transmural fibrosis. Nat Commun. 2021;12(1):5558. doi:10.1038/s41467-021-25730-0
25. Whitaker J, Bredfeldt J, Williams SE, et al. Ventricular conduction velocity following multimodal ablation including stereotactic body radiation therapy for refractory ventricular tachycardia. JACC Clin Electrophysiol. 2023;9(1):119-121. doi:10.1016/j.jacep.2022.08.032
26. Campos FO, Neic A, Mendonca Costa C, et al. An automated near-real time computational method for induction and treatment of scar-related ventricular tachycardias. Med Image Anal. 2022;80:102483. doi:10.1016/j.media.2022.102483