ADVERTISEMENT
Advanced Cardiovascular Imaging in Ventricular Arrhythmia
Ventricular arrhythmia (VA) is a significant cause of morbidity and mortality worldwide. It is a leading mechanism of sudden cardiac death (SCD), which accounts for 300,000-400,000 deaths per year.1 In ischemic cardiomyopathy (ICM), functional and structural reentry occurs within ischemic and fibrotic myocardium precipitated by coronary artery disease (CAD).
Ventricular tachycardia (VT) or ventricular fibrillation precipitated by R-on-T phenomena can propagate through these reentrant circuits. On the other hand, nonischemic cardiomyopathy (NICM) describes a panoply of cardiac diseases, including genetic and infiltrative that can, via alternate mechanisms, lead to fibrosis and remodeling, also a substrate for VA. Clinically, primary prevention of VA is accomplished with an implantable cardioverter-defibrillator (ICD) and medical optimization, with different criteria in secondary prevention. These criteria include clinical signs of heart failure and imaging evidence of decreased ejection fraction (EF) <35%.2 However, the value of imaging in the assessment and prediction of VA extends beyond solely measuring EF, as we seek to describe in this article.
Advanced cardiac imaging includes the modalities of computed tomography (CT), cardiac magnetic resonance imaging (CMR), positron emission tomography (PET), and single-photon emission computerized tomography (SPECT). These promise a noninvasive means of risk stratification by characterization of the myocardial substrate. Perhaps the most prominent example is late gadolinium enhancement (LGE) on CMR, which corresponds to myocardial fibrosis, and potentially, foci of reentry. Similarly, CT has been used to assess myocardial fat and wall thinning, which has also been linked with reentry. Finally, the utility of PET and SPECT has varied widely from the study of viability and innervation to inflammation. This article will focus predominantly on recent developments in CMR and CT.
Cardiac Magnetic Resonance
LGE in ICM
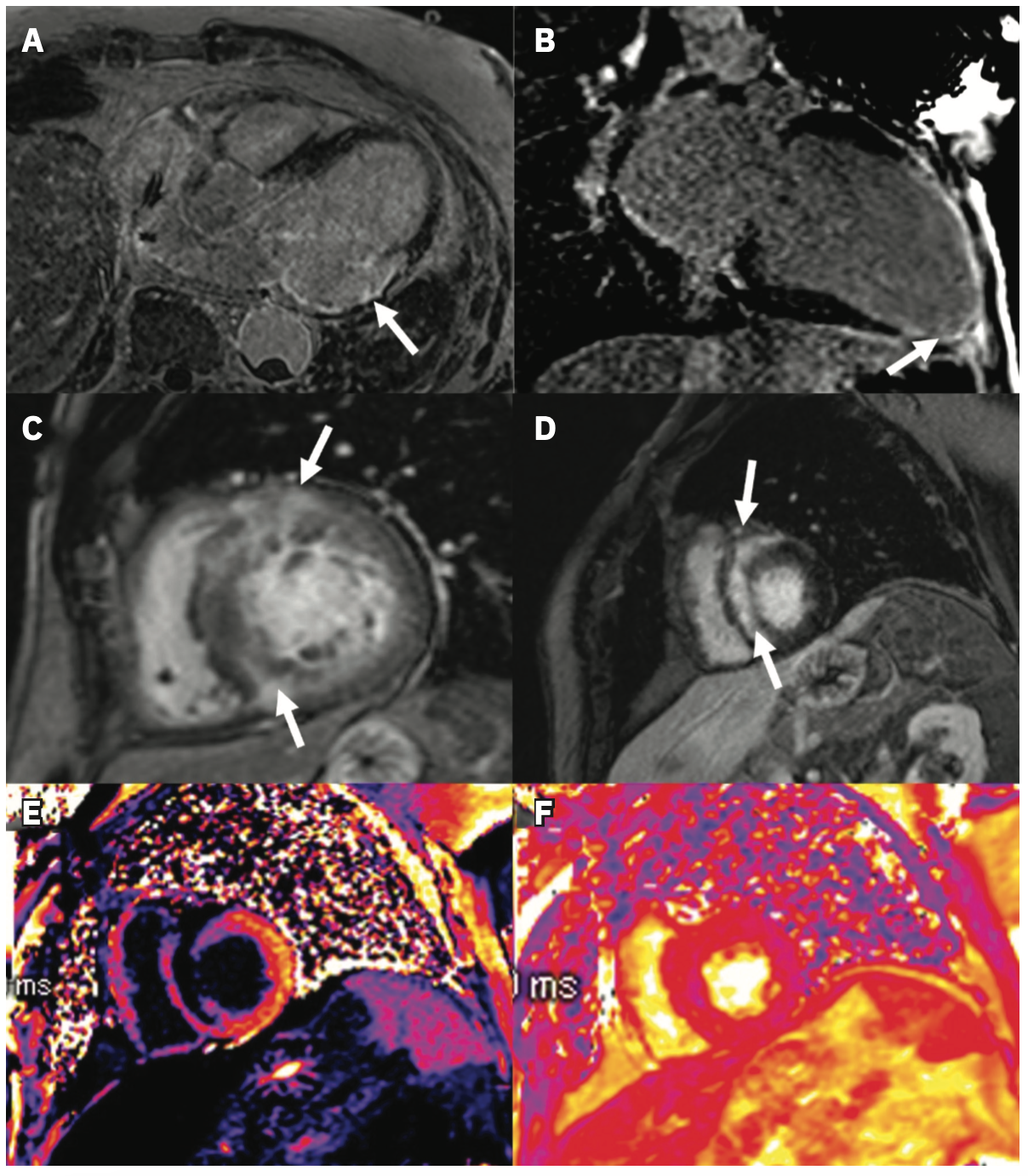
LGE assessment is well-established in clinical practice, particularly as a diagnostic tool, and its association with VA is widely described.3,4 We include examples of LGE identified in patients with ICM (Figure 1A, 1B) and NICM (Figure 1C, 1D). Several studies among patients with ICM and ICDs have shown that characteristics of LGE based on regions of heterogeneous scar termed gray zone (GZ) predict VA after controlling for comorbidities and patient level factors.5-7 Recently, it has been demonstrated that this association is not unique to low EF cohorts; among patients with an average EF of 46%, GZ mass was a better predictor of SCD than left ventricular (LV) EF.8 More complex assessments of scar and GZ have also been developed, such as LV entropy, a means of quantifying the distribution of pixel intensity of LGE.9,10 Entropy may have a stronger association with outcomes than the presence or mass of LGE and is perhaps a quantitatively richer metric.10,11 More directly regarding the outcome, LGE has also been used to identify conducting channels in CAD, mechanistically central to VA sustainability.12 Notably, this was also applicable in a mixed ICM/NICM cohort, wherein the presence of these channels and their mass was associated with VA risk.13 An example of CMR with associated electroanatomic voltage mapping data is shown in Figure 2.
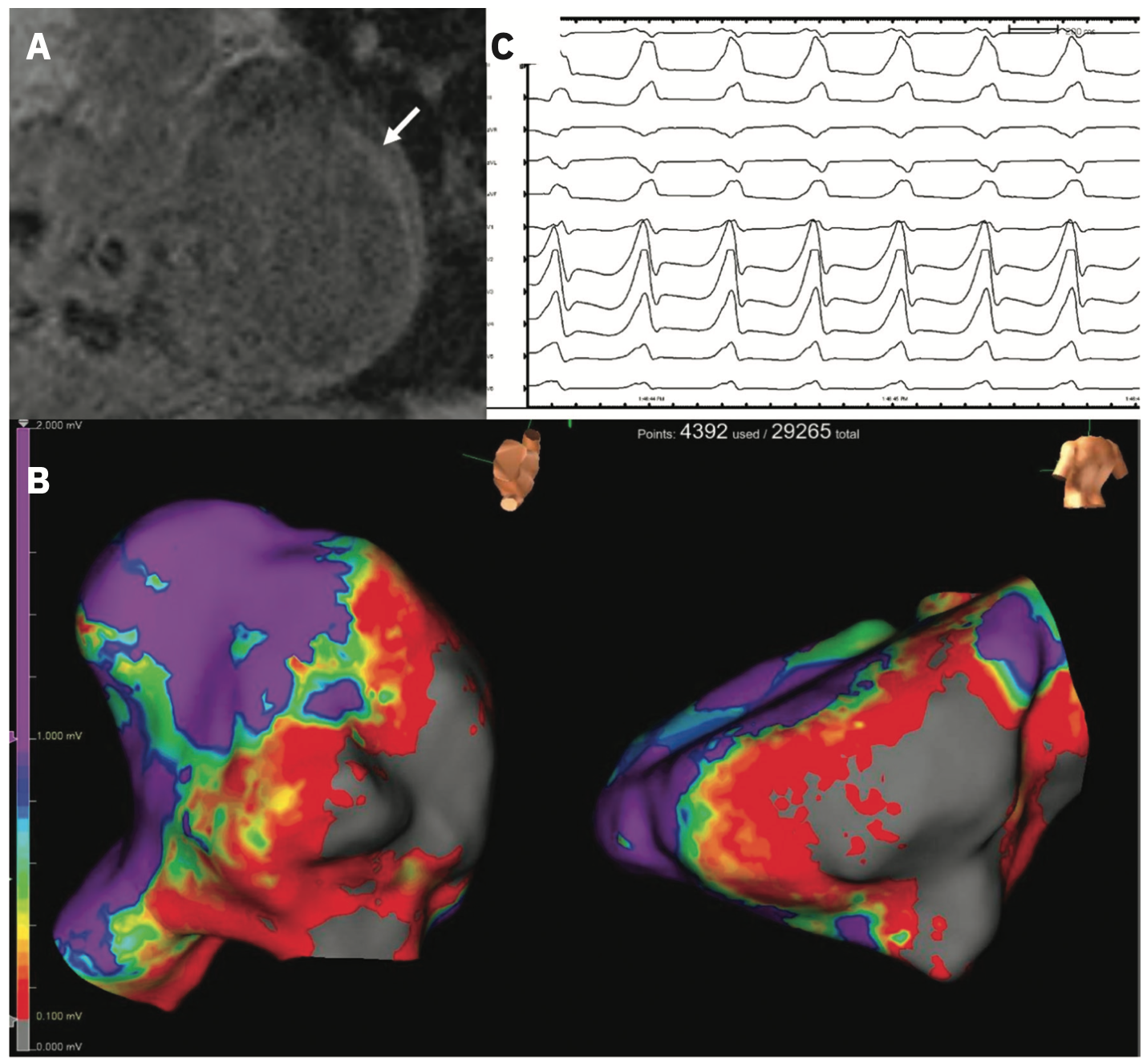
Of greater interest to general cardiologists may be that the absence of LGE has been used to identify subsets of patients with particularly low risk of VA.14 This has its parallel in the incorporation of the absence of coronary artery calcium (CAC) as a strong negative predictor of cardiovascular events.15 The utility of LGE for arrhythmic risk stratification is further supported by a random forest survival analysis in which scar and GZ mass by LGE were identified as top predictors of VA.16 In addition, serum biomarkers have been incorporated into such models, wherein a subgroup of patients with low high-sensitivity C-reactive protein and low GZ mass on LGE were identified to be at the lowest risk for VA.17
LGE in NICM
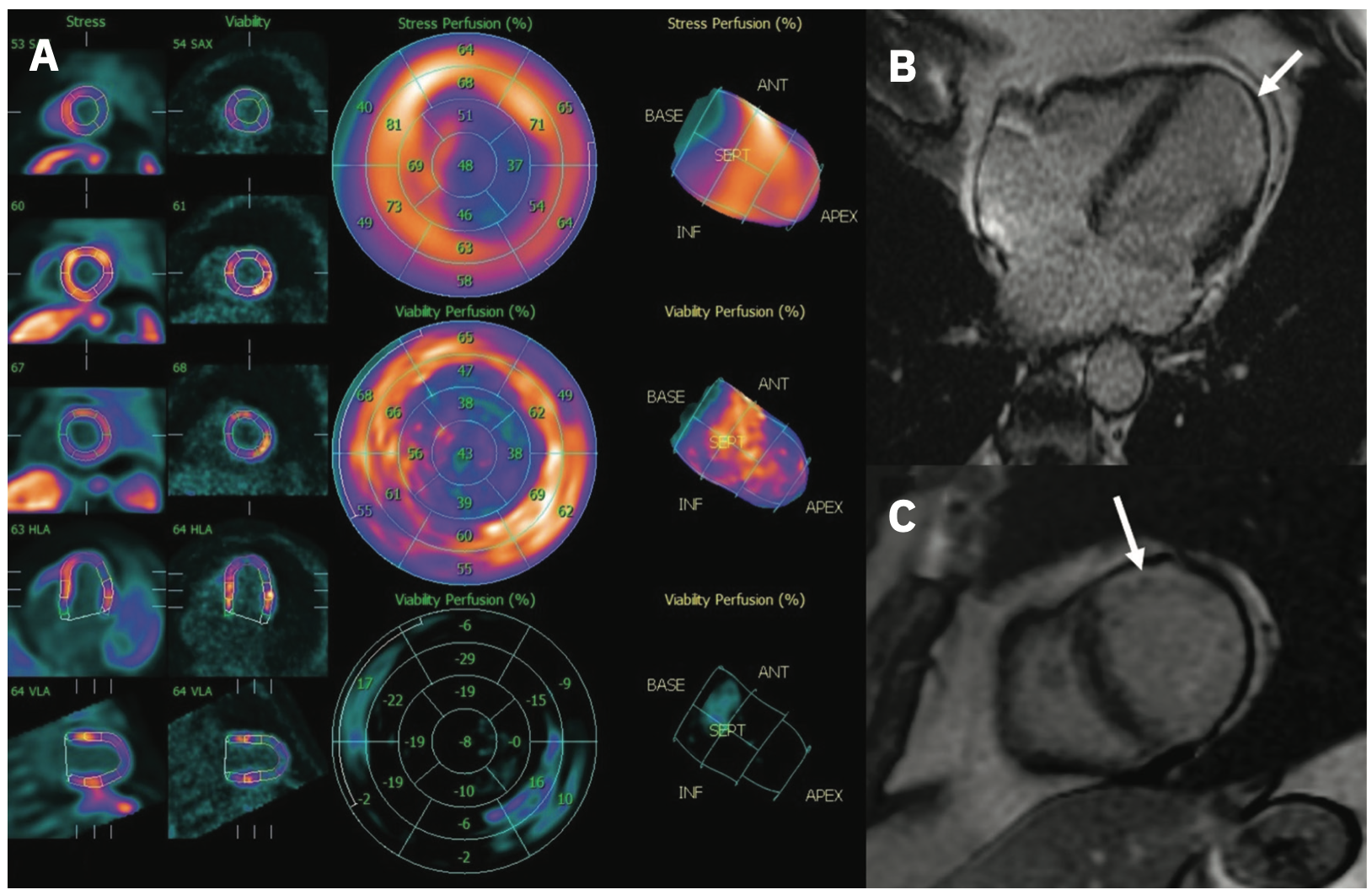
Because of the sheer breadth of cardiovascular diseases comprising NICM, many studies of LGE among these patients include a composite of phenotypes and etiologies.18-20 Particular attention has been paid to patients with NICM who may not meet EF criteria for primary prevention ICD. In dilated cardiomyopathy (DCM), for example, the presence of mid-wall LGE among patients with EF >40% was associated with a higher risk for SCD or aborted SCD.21 Among patients with hypertrophic cardiomyopathy (HCM), LGE extent has, in some cases, exceeded the performance of traditional clinical risk models, including EF.22-24 Along these lines, proposals have been made to define LGE thresholds as a means of risk stratification. LGE extent >10% identified HCM patients with an order of magnitude greater risk of VA and SCD than existing clinical risk scores suggest.25 The value of LGE in prognostication has further been illustrated among cohorts where EF may not be significantly affected by disease progression. DCM patients with progression of fibrosis as detected by serial LGE imaging had, on average, minimal change in EF (<5%).26 This subgroup was at considerably higher risk for all-cause mortality than those without fibrotic progression. It should be noted that while LGE has not yet been incorporated into clinical guidelines, there are several randomized control trials underway that will further elucidate the role of LGE in cardiologists’ daily practice.4,18 We include an example of a patient imaged with both CMR and PET to illustrate how multimodality assessment may also play a role in the future (Figure 3).
Shortcomings of LGE and Other CMR
Though LGE is the most prominent feature of CMR for VA risk stratification, other sequences may be able to address some of its shortcomings. For example, LGE-CMR relies on the correct timing of contrast injection and appropriate selection of scanning parameters, which can confound the interpretation of resultant images when suboptimal. In addition, a well-known risk of gadolinium administration is its toxicity. While modern gadolinium agents have largely done away with nephrogenic systemic fibrosis, recent consensus documents recommend shared decision-making in gadolinium administration for patients with renal impairment.27
Similar to how a moderately reduced or preserved EF does not fully encapsulate patients’ risk, there are also patients without LGE who nonetheless develop VA.28 Other CMR measures, including extracellular volume (ECV) (Figure 1E) and native T1 mapping, may prove useful for these and cardiomyopathy patients at large. Compared to LGE identifying localized fibrosis, ECV and native T1 are measures of diffuse fibrosis.29,30 In a study of DCM patients, of whom 73% had no LGE on CMR, native T1 was associated with all-cause mortality independent of LGE extent and EF.31 A smaller study specifically among HCM patients without LGE demonstrated a similar association of native T1 with SCD.32 Interestingly, in a mixed cohort of ICM and NICM, while native T1 was independently associated with VA, a measure based on GZ thresholds (ie, LGE) was more strongly associated with the outcome.33 For completion, we mention T2* mapping (Figure 1F), which has typically been used to identify pathologic iron accumulation in storage diseases but has recently been applied to fibrosis assessment.34-36 Further research is warranted to determine the role of T2* mapping for VA risk prediction.
Computed Tomography
Compared with CMR, CT is more widely available and typically lower cost for patients and payers, increasing its accessibility. Its spatial resolution is superior to CMR as a modality, though it is more limited in tissue characterization.37 The role of CT for VA risk stratification has historically been via assessment of the coronaries, whether as a CAC score or definition of anatomy.38 Beyond this, cardiac CT with delayed iodinated contrast enhancement has been used to detect fibrosis, which has been validated in specific phenotypes against LGE on CMR and even histology.39,40 In HCM, fibrotic mass detected by CT was associated with VA after adjustment for clinical risk factors.41 Particularly pertinent to the electrophysiologist, myocardial wall thickness on CT has also been used to identify channels that could represent VT isthmuses in post-myocardial infarction patients, using the modality’s high resolution (eg, the MUSIC platform, Liryc, Université de Bordeaux, Bordeaux, France, and INRIA, Sophia Antipolis, France).42 Compared with the gold standard, EP mapping of isthmuses, CT channels were 100% sensitive, albeit with a 50% positive predictive value. Along similar lines, CT has been used to characterize myocardial fat deposition, which has been observed to signal regions of infarct in ICM and, ergo, potential VT circuit sites.43 An example is shown in Figure 4. In arrhythmogenic right ventricular cardiomyopathy, characterized by fatty replacement of the myocardium, contrast-enhanced CT attenuation was closely associated with mapped conduction velocity and suggested a potential role of CT in guiding ablation.44 This association was also observed in postinfarct patients, a considerably broader population.45
Conclusion
We have described several advanced imaging modalities for risk stratification of VAs and examples of their applications, especially in CMR and CT. In coming years, these may become better established in clinical guidelines and make their way into routine practice. As a result, cardiologists and electrophysiologists in the future will likely have an expanded toolset for determining patients’ risks and appropriately intervening.
Disclosures: The authors have completed and returned the ICMJE Form for Disclosure of Potential Conflicts of Interest. Dr Xie has no conflicts of interest to report regarding the content herein. Dr Chrispin reports consulting/honorarium from Biosense Webster and Abbott.
References
1. Kuriachan VP, Sumner GL, Mitchell LB. Sudden cardiac death. Curr Probl Cardiol. 2015;40(4):133-200. doi:10.1016/j.cpcardiol.2015.01.002
2. Al-Khatib SM, Stevenson WG, Ackerman MJ, et al. 2017 AHA/ACC/HRS guideline for management of patients with ventricular arrhythmias and the prevention of sudden cardiac death: a report of the American College of Cardiology/American Heart Association task force on clinical practice guidelines and the Heart Rhythm Society. J Am Coll Cardiol. 2018;72(14):e91-e220. doi:10.1016/j.jacc.2017.10.054
3. Wu KC, Weiss RG, Thiemann DR, et al. Late gadolinium enhancement by cardiovascular magnetic resonance heralds an adverse prognosis in non-ischemic cardiomyopathy. J Am Coll Cardiol. 2008;51(25):2414-2421. doi:10.1016/j.jacc.2008.03.018
4. Wu KC. Sudden cardiac death substrate imaged by magnetic resonance imaging: from investigational tool to clinical applications. Circ Cardiovasc Imaging. 2017;10(7):e005461. doi:10.1161/CIRCIMAGING.116.005461
5. Schmidt A, Azevedo CF, Cheng A, et al. Infarct tissue heterogeneity by magnetic resonance imaging identifies enhanced cardiac arrhythmia susceptibility in patients with left ventricular dysfunction. Circulation. 2007;115(15):2006-2014. doi:10.1161/CIRCULATIONAHA.106.653568
6. Roes SD, Borleffs CJW, Geest RJvd, et al. Infarct tissue heterogeneity assessed with contrast-enhanced MRI predicts spontaneous ventricular arrhythmia in patients with ischemic cardiomyopathy and implantable cardioverter-defibrillator. Circ Cardiovasc Imaging. 2009;2(3):183-190. doi:10.1161/CIRCIMAGING.108.826529
7. Zeidan-Shwiri T, Yang Y, Lashevsky I, et al. Magnetic resonance estimates of the extent and heterogeneity of scar tissue in ICD patients with ischemic cardiomyopathy predict ventricular arrhythmia. Heart Rhythm. 2015;12(4):802-808. doi:10.1016/j.hrthm.2015.01.007
8. Zegard A, Okafor O, de Bono J, et al. Myocardial fibrosis as a predictor of sudden death in patients with coronary artery disease. J Am Coll Cardiol. 2021;77(1):29-41. doi:10.1016/j.jacc.2020.10.046
9. Muthalaly RG, Kwong RY, John RM, et al. Left ventricular entropy is a novel predictor of arrhythmic events in patients with dilated cardiomyopathy receiving defibrillators for primary prevention. JACC Cardiovasc Imaging. 2019;12(7 Pt 1):1177-1184. doi:10.1016/j.jcmg.2018.07.003
10. Androulakis AFA, Zeppenfeld K, Paiman EHM, et al. Entropy as a novel measure of myocardial tissue heterogeneity for prediction of ventricular arrhythmias and mortality in post-infarct patients. JACC Clin Electrophysiol. 2019;5(4):480-489. doi:10.1016/j.jacep.2018.12.005
11. Gould J, Porter B, Claridge S, et al. Mean entropy predicts implantable cardioverter-defibrillator therapy using cardiac magnetic resonance texture analysis of scar heterogeneity. Heart Rhythm. 2019;16(8):1242-1250. doi:10.1016/j.hrthm.2019.03.001
12. Perez-David E, Arenal Á, Rubio-Guivernau JL, et al. Noninvasive identification of ventricular tachycardia-related conducting channels using contrast-enhanced magnetic resonance imaging in patients with chronic myocardial infarction. J Am Coll Cardiol. 2011;57(2):184-194. doi:10.1016/j.jacc.2010.07.043
13. Sánchez-Somonte P, Quinto L, Garre P, et al. Scar channels in cardiac magnetic resonance to predict appropriate therapies in primary prevention. Heart Rhythm. 2021;18(8):1336-1343. doi:10.1016/j.hrthm.2021.04.017
14. Leyva F, Zegard A, Okafor O, et al. Myocardial fibrosis predicts ventricular arrhythmias and sudden death after cardiac electronic device implantation. J Am Coll Cardiol. 2022;79(7):665-678. doi:10.1016/j.jacc.2021.11.050
15. Budoff MJ, McClelland RL, Nasir K, et al. Cardiovascular events with absent or minimal coronary calcification: the Multi-Ethnic Study of Atherosclerosis (MESA). Am Heart J. 2009;158(4):554-561. doi:10.1016/j.ahj.2009.08.007
16. Wu KC, Wongvibulsin S, Tao S, et al. Baseline and dynamic risk predictors of appropriate implantable cardioverter defibrillator therapy. J Am Heart Assoc. 2020;9(20):e017002. doi:10.1161/JAHA.120.017002
17. Wu KC, Gerstenblith G, Guallar E, et al. Combined cardiac magnetic resonance imaging and C-reactive protein levels identify a cohort at low risk for defibrillator firings and death. Circ Cardiovasc Imaging. 2012;5(2):178-186. doi:10.1161/CIRCIMAGING.111.968024
18. Ganesan AN, Gunton J, Nucifora G, McGavigan AD, Selvanayagam JB. Impact of late gadolinium enhancement on mortality, sudden death and major adverse cardiovascular events in ischemic and non-ischemic cardiomyopathy: a systematic review and meta-analysis. Int J Cardiol. 2018;254:230-237. doi:10.1016/j.ijcard.2017.10.094
19. Di Marco A, Anguera I, Schmitt M, et al. Late gadolinium enhancement and the risk for ventricular arrhythmias or sudden death in dilated cardiomyopathy: systematic review and meta-analysis. JACC: Heart Fail. 2017;5(1):28-38. doi:10.1016/j.jchf.2016.09.017
20. Kuruvilla S, Adenaw N, Katwal AB, et al. Late gadolinium enhancement on cardiac magnetic resonance predicts adverse cardiovascular outcomes in non-ischemic cardiomyopathy: a systematic review and meta-analysis. Circ Cardiovasc Imaging. 2014;7(2):250-258. doi:10.1161/CIRCIMAGING.113.001144
21. Halliday BP, Gulati A, Ali A, et al. Association between midwall late gadolinium enhancement and sudden cardiac death in patients with dilated cardiomyopathy and mild and moderate left ventricular systolic dysfunction. Circulation. 2017;135(22):2106-2115. doi:10.1161/CIRCULATIONAHA.116.026910
22. Greulich S, Seitz A, Herter D, et al. Long-term risk of sudden cardiac death in hypertrophic cardiomyopathy: a cardiac magnetic resonance outcome study. Eur Heart J Cardiovasc Imaging. 2021;22(7):732-741. doi:10.1093/ehjci/jeaa423
23. Rowin EJ, Maron MS, Adler A, et al. Importance of newer cardiac magnetic resonance-based risk markers for sudden death prevention in hypertrophic cardiomyopathy: an international multicenter study. Heart Rhythm. 2022;19(5):782-789. doi:10.1016/j.hrthm.2021.12.017
24. O’Mahony C, Jichi F, Pavlou M, et al. A novel clinical risk prediction model for sudden cardiac death in hypertrophic cardiomyopathy (HCM risk-SCD). Eur Heart J. 2014;35(30):2010-2020. doi:10.1093/eurheartj/eht439
25. Todiere G, Nugara C, Gentile G, et al. Prognostic role of late gadolinium enhancement in patients with hypertrophic cardiomyopathy and low-to-intermediate sudden cardiac death risk score. Am J Cardiol. 2019;124(8):1286-1292. doi:10.1016/j.amjcard.2019.07.023
26. Mandawat A, Chattranukulchai P, Mandawat A, et al. Progression of myocardial fibrosis in nonischemic dcm and association with mortality and heart failure outcomes. JACC Cardiovasc Imaging. 2021;14(7):1338-1350. doi:10.1016/j.jcmg.2020.11.006
27. Weinreb JC, Rodby RA, Yee J, et al. Use of intravenous gadolinium-based contrast media in patients with kidney disease: consensus statements from the American College of Radiology and the National Kidney Foundation. Kidney Med. 2021;3(1):142-150. doi:10.1016/j.xkme.2020.10.001
28. Voskoboinik A, Wong MCG, Elliott JK, et al. absence of late gadolinium enhancement on cardiac magnetic resonance imaging in ventricular fibrillation and non-ischemic cardiomyopathy. Pacing Clin Electrophysiol. 2018;41(9):1109-1115. doi:10.1111/pace.13426
29. Kammerlander AA, Marzluf BA, Zotter-Tufaro C, et al. T1 mapping by CMR imaging: from histological validation to clinical implication. JACC Cardiovasc Imaging. 2016;9(1):14-23. doi:10.1016/j.jcmg.2015.11.002
30. Messroghli DR, Moon JC, Ferreira VM, et al. Clinical recommendations for cardiovascular magnetic resonance mapping of T1, T2, T2* and extracellular volume: a consensus statement by the Society for Cardiovascular Magnetic Resonance (SCMR) endorsed by the European Association for Cardiovascular Imaging (EACVI). J Cardiovasc Magn Reson. 2017;19(1):1-24. doi:10.1186/s12968-017-0389-8
31. Puntmann VO, Carr-White G, Jabbour A, et al. T1-mapping and outcome in nonischemic cardiomyopathy: all-cause mortality and heart failure. JACC Cardiovasc Imaging. 2016;9(1):40-50. doi:10.1016/j.jcmg.2015.12.001
32. Xu J, Zhuang B, Sirajuddin A, et al. MRI T1 mapping in hypertrophic cardiomyopathy: evaluation in patients without late gadolinium enhancement and hemodynamic obstruction. Radiology. 2020;294(2):275-286. doi:10.1148/radiol.2019190651
33. Chen Z, Sohal M, Voigt T, et al. Myocardial tissue characterization by cardiac magnetic resonance imaging using T1 mapping predicts ventricular arrhythmia in ischemic and non-ischemic cardiomyopathy patients with implantable cardioverter-defibrillators. Heart Rhythm. 2015;12(4):792-801. doi:10.1016/j.hrthm.2014.12.020
34. Triadyaksa P, Oudkerk M, Sijens PE. Cardiac T2* mapping: techniques and clinical applications. J Magn Reson Imaging. 2020;52(5):1340-1351. doi:10.1002/jmri.27023
35. Gastl M, Gotschy A, von Spiczak J, et al. Cardiovascular magnetic resonance T2* mapping for structural alterations in hypertrophic cardiomyopathy. Eur J Radiol Open. 2019;6:78-84. doi:10.1016/j.ejro.2019.01.007
36. Gastl M, Gruner C, Labucay K, et al. Cardiovascular magnetic resonance T2* mapping for the assessment of cardiovascular events in hypertrophic cardiomyopathy. Open Heart. 2020;7(1):e001152. doi:10.1136/openhrt-2019-001152
37. Mahida S, Sacher F, Dubois R, et al. Cardiac imaging in patients with ventricular tachycardia. Circulation. 2017;136(25):2491-2507. doi:10.1161/CIRCULATIONAHA.117.029349
38. Sparrow P, Merchant N, Provost Y, et al. Cardiac MRI and CT features of inheritable and congenital conditions associated with sudden cardiac death. Eur Radiol. 2009;19(2):259-270. doi:10.1007/s00330-008-1169-5
39. Langer C, Lutz M, Eden M, et al. Hypertrophic cardiomyopathy in cardiac CT: a validation study on the detection of intramyocardial fibrosis in consecutive patients. Int J Cardiovasc Imaging. 2014;30(3):659-667. doi:10.1007/s10554-013-0358-8
40. Bandula S, White SK, Flett AS, et al. Measurement of myocardial extracellular volume fraction by using equilibrium contrast-enhanced CT: validation against histologic findings. Radiology. 2013;269(2):396-403. doi:10.1148/radiology.13130130
41. Shiozaki AA, Senra T, Arteaga E, et al. Myocardial fibrosis detected by cardiac CT predicts ventricular fibrillation/ventricular tachycardia events in patients with hypertrophic cardiomyopathy. J Cardiovasc Comput Tomogr. 2013;7(3):173-181. doi:10.1016/j.jcct.2013.04.002
42. Takigawa M, Duchateau J, Sacher F, et al. Are wall thickness channels defined by computed tomography predictive of isthmuses of postinfarction ventricular tachycardia? Heart Rhythm. 2019;16(11):1661-1668. doi:10.1016/j.hrthm.2019.06.012
43. Sasaki T, Calkins H, Miller CF, et al. New insight into scar-related ventricular tachycardia circuits in ischemic cardiomyopathy: fat deposition after myocardial infarction on computed tomography--a pilot study. Heart Rhythm. 2015;12(7):1508-1518. doi:10.1016/j.hrthm.2015.03.041
44. Ustunkaya T, Desjardins B, Wedan R, et al. Epicardial conduction speed, electrogram abnormality, and computed tomography attenuation associations in arrhythmogenic right ventricular cardiomyopathy. JACC Clin Electrophysiol. 2019;5(10):1158-1167. doi:10.1016/j.jacep.2019.06.017
45. Ustunkaya T, Desjardins B, Liu B, et al. Association of regional myocardial conduction velocity with the distribution of hypoattenuation on contrast-enhanced perfusion computed tomography in patients with postinfarct ventricular tachycardia. Heart Rhythm. 2019;16(4):588-594. doi:10.1016/j.hrthm.2018.10.029