Emerging Technologies and Innovation in Cardiac Electrophysiology: From Benchtop to Bedside
Background
In the 1780s, the findings of Luigi Galvani laid the foundations of cardiac electrophysiology (EP). Galvani found that applying an electric shock to the nerve on a dead frog’s leg could cause twitching of the leg’s muscle. He hypothesized that animal tissue has intrinsic electricity, which is responsible for activating nerves and muscles.1 In 1887, the first human electrocardiogram (ECG) was recorded by Augustus Waller with a capillary electrometer.2 By mathematical correction and evaluation of the capillary electrometer curves, Dr Willem Einthoven recognized 5 deflections of electrical current passing through the heart during the cardiac cycle. He named these P, Q, R, S, and T, and used “electrocardiogram” to name the graphical recording of the cardiac cycle.3 Dr Einthoven invented the high-sensitive string galvanometer in 1901 and the first practical ECG in 1903.4 The first use of electrocardiography in clinical medicine was credited to Sir Thomas Lewis in 1908.5 Dr Lewis and Dr Arthur MacNulty published a joint paper in 1908 describing the first use of the electrocardiograph for the diagnosis of heart disease.6 Dr Lewis studied other cardiac rhythm abnormalities such as atrial flutter, atrial fibrillation (AF), and atrial and bundle branch block, and defined the mechanisms and clinical features of each.7 In 1913, he published Clinical Electrocardiography, which is considered to be the first book published on the subject.7 Later, Dr Lewis resumed his study of arrhythmias and published his findings in 1920 in The Mechanism and Graphic Registration of the Heart Beat.8 After 1920, further advancement in electrocardiographic knowledge was achieved by Dr Frank N. Wilson via his group studies. In 1944, Dr Wilson and colleagues described the role of unipolar precordial leads in clinical cardiology, and introduced precordial leads to measure the potentials close to the heart. This was the beginning of electrocardiography in its present form.8 Dr Dirk Durrer was also a true pioneer of clinical electrophysiology. He invented the multiterminal intramural needle electrode in the early 1960s, which opened the door to programmed electrical stimulation and recording of the human heart.9 In 1967, two independent groups studied the mechanism and source of arrhythmia in the intact human heart by use of programmed electric stimulation and intracardiac activation mapping: Durrer et al in patients with Wolff-Parkinson-White syndrome, and Coumel et al in a patient with atrioventricular junctional tachycardia.10 These studies could be considered the origin of clinical electrophysiology.2 Significant progress has also been made during the past 40 years in the diagnosis and treatment of cardiac arrhythmias.
Over the past 135 years, marvelous progress in the diagnosis and treatment of cardiac arrhythmias has enabled us to possibly cure many patients with arrhythmias. At the Electrophysiology Clinical Research and Innovations (EPCRI) department at the Texas Heart Institute, we work on different research projects to expand our knowledge of arrhythmias and improve patient management. These projects include ex vivo studies, machine learning studies, animal studies, and human subject studies. We have a unique capacity to design and test new devices to address the needs of patients and physicians, which leads to enhanced procedural outcomes and patient safety. This paper aims to briefly introduce our latest projects and share an update on our ongoing studies.
Catheter Lab on a Bench for Ex Vivo Testing
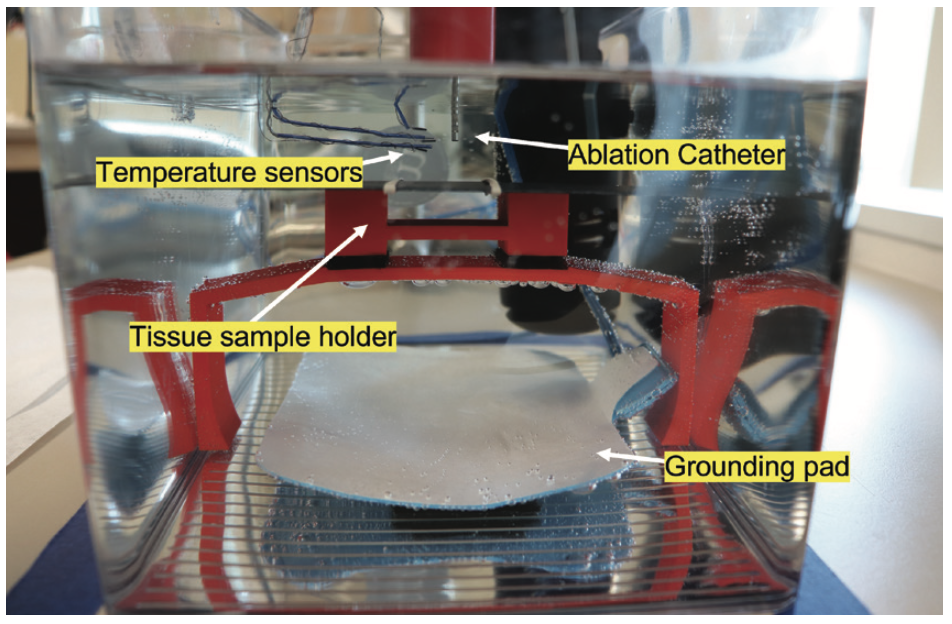
The EPCRI lab has developed a modular ex vivo testing setup for iteratively developing and testing catheters as well as studying the underlying biophysics of ablation (Figure 1). The team used this ex vivo test setup to better understand the effects of atrial ablation on esophageal lesion formations, specifically, the changes in luminal and esophageal surface temperature during standard power (10/20/30 W) and high power, short duration (HPSD) (40/45/50 W) ablation. We used fresh porcine hearts and esophageal sections. The study showed that after ablation, the esophageal luminal temperature peaked after a certain delay (HSPD 24.2 ± 22.1 sec vs Standard 13.0 ± 11.0 sec; P=.023). The rise in epiesophageal and endoluminal temperature was greater in HPSD compared to standard ablation (epiesophageal 5.9 ± 5.6 vs 2.2 ± 2.0°C; P<.01; endoluminal 0.7 ± 0.5 vs 0.4 ± 0.2°C; P<.01). Furthermore, the study found that endoluminal temperature readings significantly underestimate the temperature on the surface of the esophagus during HPSD.11
Improved Clinical Care Through Real-time Applications of Machine Learning

The EPCRI lab, in collaboration with the Department of Electrical and Computer Engineering at Rice University, has developed an algorithm that can compute 12-lead ECGs from intracardiac electrogram (EGM) data. Our goal was to propose and implement a method for making a standard 12-lead ECG from a set of EGM leads. We created a nonlinear function between images of different channel numbers via our novel convolutional neural network (CNN) model. The algorithm uses a nonlinear regression with a convolutional encoder-decoder for converting the dataset. The algorithm works in a patient-specific manner by utilizing short time series data obtained from the individual patient to set coefficients (Figure 2). In addition, we tested the model in the reverse direction to reconstruct EGMs from a 12-lead ECG and achieved high reconstruction accuracy.12 In a retrospective diagnostic EP study of 14 patients, concurrent EGM and 12-lead ECG signals were used to compute the transformation from EGM to ECG, and vice versa. The signals were separated into discrete time blocks containing a single heartbeat. The data blocks were converted into the time-frequency domain to produce image-like spectrograms. These were then fed into a convolutional encoder-decoder neural network model—a specific type of CNN—to learn a function between EGM and ECG signals. For each patient, the algorithm was trained on a short training dataset from 14 patients and validated on the remaining dataset per patient. This allowed for the computation of the 12-lead ECG of previously recorded arrhythmic episodes after training the algorithm for a short amount of time in an inpatient setting. Initially, EGM data from 5 channels and then 1 channel (the EGM data were obtained from a diagnostic catheter placed in the coronary sinus) were used to reconstruct 12-lead ECGs in 14 patients. The average Pearson correlation coefficient was above 0.9 for all patients. The reverse problem of reconstructing 5 EGM leads from a 12-lead ECG was also shown, with a correlation coefficient above 0.9 for most patients.
Using a novel method termed RT-RCG (Real-Time and high-quality Reconstruction of ECG signals from EGM), the algorithm can be realized in energy-efficient hardware to compute 12-lead ECG signals in real time from electrograms. This method automatically selects an optimal neural network and corresponding accelerator to catalyze the hardware development process.13 The algorithm used in a small clinical dataset showed the ability to choose an appropriate network from 9 encoder-decoder networks to compute ECG signals from a single EGM channel with a correlation of 0.942 to 0.983 in a patient-specific manner.14 This study has been supported in part by the National Science Foundation and the National Institutes of Health (NIH).
Injectable Electrodes to Treat Ventricular Arrhythmias
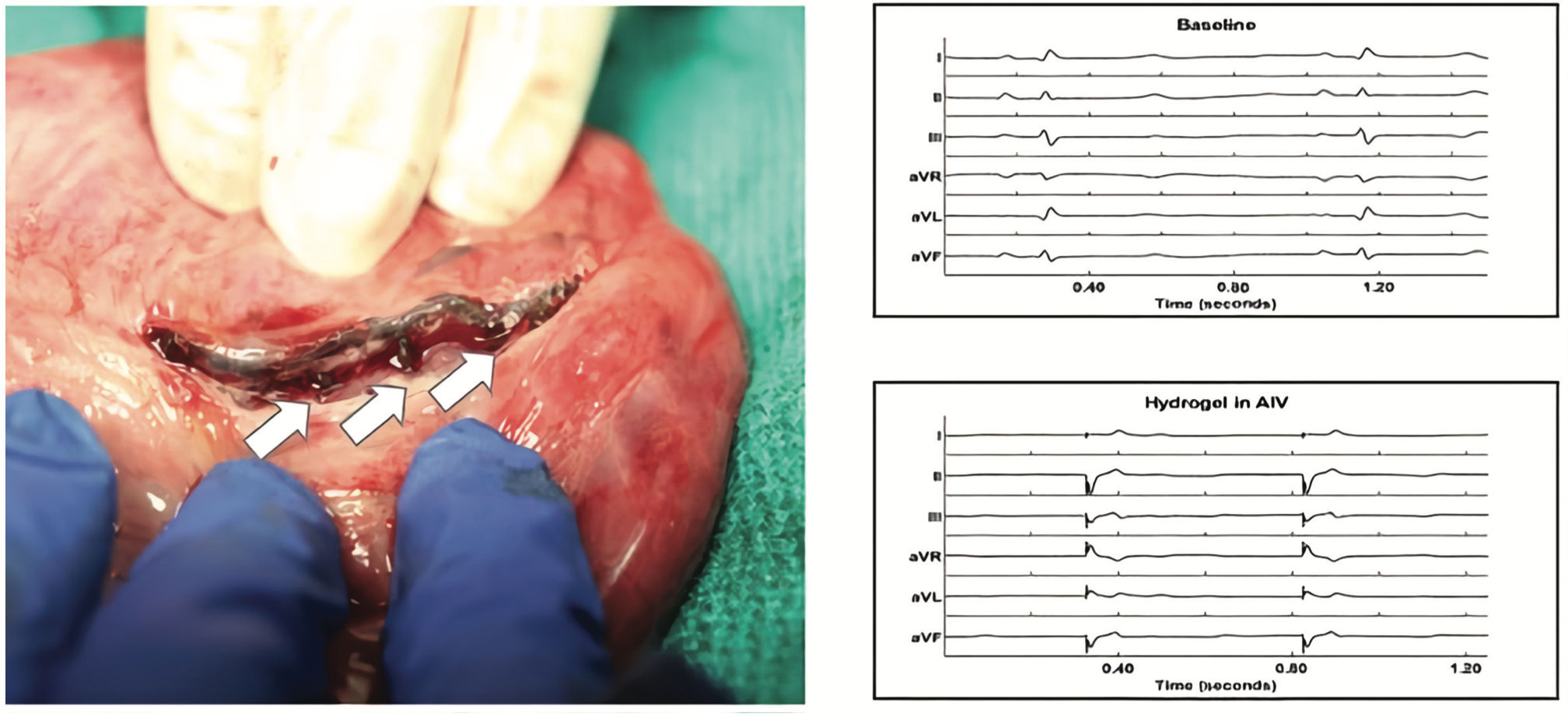
The EPCRI lab, in collaboration with the Cosgriff-Hernandez Lab at the University of Texas at Austin, has created injectable hydrogel electrodes that can be used to provide novel pacing therapies (Figure 3). Because of their size, current lead systems are limited to placement in large vessels, and this is a limitation in their recruitment potential. An injectable conductive hydrogel, which cures in situ to fill a venous branch, may provide a more robust pacing response by enabling greater myocardial recruitment. Over the last year, the lab tested out a formulation of an in situ curing conductive hydrogel that can be delivered into a venous branch. We have performed a series of acute in vivo studies in porcine models and have shown that the injected hydrogel can capture tissue in the mid-myocardial region. In this study, we injected the hydrogel with a custom catheter system through an 18G sheath into the anterior interventricular vein. A temporary pacing needle was inserted into the gel for stimulation. We were able to pace the heart and record heart activities through the injected hydrogel. The capture of the mid-myocardium paves the way to the possibility of creating patient-specific electrodes for improving capture. This work was presented at Heart Rhythm 2021.14
Wireless Pacing System for Improved Cardiac Resynchronization Therapy

Our lab is developing a wirelessly powered pacing system in collaboration with the Departments of Electrical and Computer Engineering at UCLA and Rice University. This effort is funded by an R01 grant from the NIH. The work has resulted in the development of a pacing network of nodes that can be programmed to have different pulse widths and delays (Figure 4). The wirelessly powered pacemaker, which features µW-level power consumption and high flexibility in a miniature size, makes it suitable for epicardial implantation. We previously published the early stages of this work,15 and since then, have made significant progress in the development of a transvenous deployable pacing node.
Safer Pericardial Access Using Bioimpedance
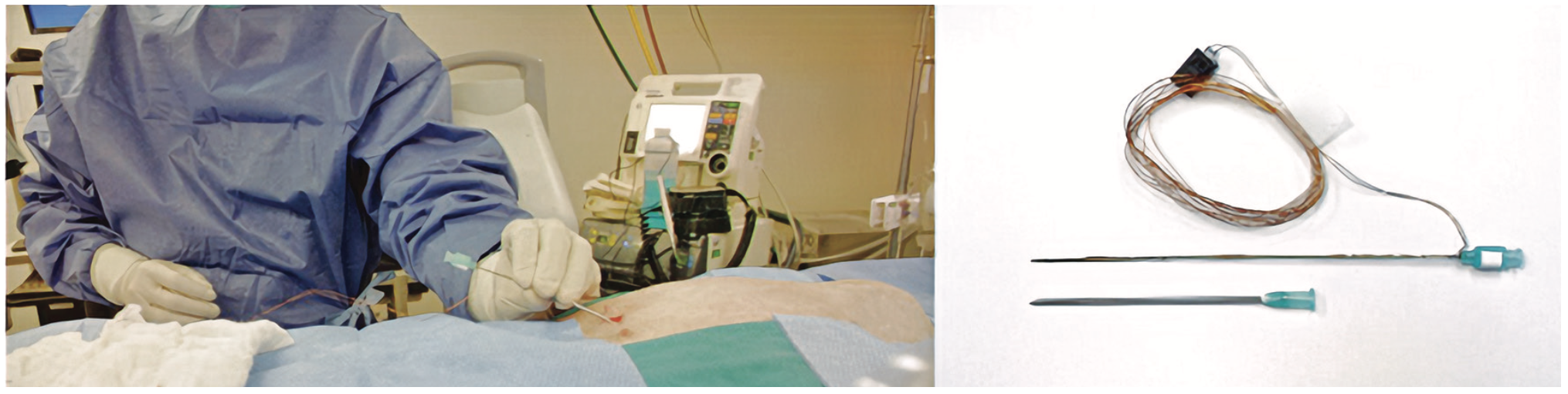
We developed a 21G custom needle that can further improve outcomes when using a micropuncture needle to access the pericardial sac (Figure 5). This device uses continuous real-time bioimpedance readings to differentiate between tissue planes as the operator inserts a needle. Every tissue plane in the path of the needle has a unique bioimpedance signature that can be used for differentiation. This allows the user to stop inserting the needle once it is in the target tissue plane. We tested this device in 3 ovine models. A significant difference was observed between average normalized impedance values of the pericardial space (1.760 ± 0.370), anterior mediastinum (3.209 ± 0.227), and right ventricle (1.024 ± 0.207; P<.0001).16 The needle could also be used for central line access procedures to reduce pneumothorax-related complications.17
Continuous Diaphragmatic Stimulation
The EPCRI lab, in collaboration with TMC Biodesign, designed an endotracheal device to stimulate the phrenic nerve in order to stimulate the diaphragm. Continuous diaphragmatic stimulation has shown significant improvement in the quality of life of patients in the intensive care unit after they are weaned. The new devices use a modified endotracheal tube with electrodes to selectively pace the phrenic nerve. This work has been supported in part by the Roderick D. MacDonald Research Fund.
Cryptogenic Stroke and AF Monitoring by Insertable Cardiac Monitor
In 2020, we evaluated the incidence of inappropriate pause detection during remote cardiac monitoring with an insertable cardiac monitor (ICM).18 This retrospective study among 242 consecutive patients with an ICM showed that 84 patients (average age 63.4, female = 38) had at least one reported pause episode. The rate of inappropriate (false-positive) pause detection was 51% (43 of 84). The study revealed that there was a high rate of inappropriate pause detection requiring further tracing examination by an expert and that more robust algorithms were needed to reduce the rate of false-positives.
We are currently working on 2 ICM-related projects. In the first, we aim to detect the rate of inappropriate AF detection with ICM. In the other project, we focus on finding the rate of AF detection with the ICM in patients with cryptogenic stroke. Limited data are available from real-world studies about the incidence of AF among these patients, and there is a notable difference between the published studies. These findings will help improve the management of patients with cryptogenic stroke.
Summary
The field of EP has witnessed significant advancements over the past 40 years. This paper offers a snapshot of some of our EP-related research projects taking place at EPCRI. We encourage readers to learn more about our projects and detailed findings from the cited papers.
Disclosures: The authors have completed and returned the ICMJE Form for Disclosure of Potential Conflicts of Interest. Dr Chelu reports grants or contracts from Abbott and Impulse Dynamics, and support for attending meetings and/or travel from Biosense Webster. Dr Mehdi Razavi and Mathews John, MEng, report patents planned, issued or pending (US20190224476A1, US20200129086A1, and WO2021046441A1); they also report stock/stock options as stakeholders of Maxwell Biomedical. The other authors have no conflicts of interest to report regarding the content herein.
References
1. Rubaiy HN. A short guide to electrophysiology and ion channels. J Pharm Pharm Sci. 2017;20:48-67. doi:10.18433/J32P6R
2. Lüderitz B. Historical perspectives of cardiac electrophysiology. Hellenic J Cardiol. 2009;50(1):3-16.
3. AlGhatrif M, Lindsay J. A brief review: history to understand fundamentals of electrocardiography. J Community Hosp Intern Med Perspect. 2012;2(1). doi:10.3402/jchimp.v2i1.14383
4. Barold SS. Willem Einthoven and the birth of clinical electrocardiography a hundred years ago. Card Electrophysiol Rev. 2003;7(1):99-104. doi:10.1023/a:1023667812925.
5. Cygankiewicz I. Sir Thomas Lewis (1881-1945). Cardiol J. 2007;14(6):605-606.
6. Plarr’s Lives of the Fellows. Royal College of Surgeons of England. September 11, 2014. Accessed January 27, 2022. https://livesonline.rcseng.ac.uk/client/en_GB/lives/search/results?qu=%22RCS: E005926%22&rt=false|||IDENTIFIER|||Resource+Identifier
7. Shapiro E. The first textbook of electrocardiography. Thomas Lewis: clinical electrocardiography. J Am Coll Cardiol. 1983;1(4):1160-1161. doi:10.1016/s0735-1097(83)80120-x
8. Fisch C. Centennial of the string galvanometer and the electrocardiogram. J Am Coll Cardiol. 2000;36(6):1737-1745. doi:10.1016/s0735-1097(00)00976-1
9. Opthof T, Janse MJ, Kléber AG, et al. The works of Dirk Durrer (1918-1984). Neth Heart J. 2012;20(10):430-433. doi:10.1007/s12471-012-0313-7
10. Wellens HJ. Forty years of invasive clinical electrophysiology: 1967-2007. Circ Arrhythm Electrophysiol. 2008;1(1):49-53. doi:10.1161/CIRCEP.108.770529
11. Kar R, Post A, John M, Rook A, Razavi M. An initial ex vivo evaluation of temperature profile and thermal injury formation on the epiesophageal surface during radiofrequency ablation. J Cardiovasc Electrophysiol. 2021;32(3):704-712. doi:10.1111/jce.14911
12. Banta A, Cosentino R, John M, et al. A novel convolutional neural network for reconstructing surface electrocardiograms from intracardiac electrograms and vice versa. Artif Intell Med. 2021;118:102135. doi:10.1016/j.artmed.2021.102135
13. Zhang Y, Banta A, Fu Y, et al. RT-RCG: Neural network and accelerator search towards effective and real-time ECG reconstruction from intracardiac electrograms. ACM J Emerg Technol Comput Syst. 2021. doi.org/10.1145/1122445.1122456
14. Buchan S, Post A, John MM, et al. B-PO04-044 Conductive system capture via in-situ curing hydrogel electrode. Heart Rhythm. 2021;18(8)(suppl):S296-S297. doi.org/10.1016/j.hrthm.2021.06.740
15. Lyu H, John M, Burkland D, et al. Synchronized biventricular heart pacing in a closed-chest porcine model based on wirelessly powered leadless pacemakers. Sci Rep. 2020;10(1):2067. doi:10.1038/s41598-020-59017-z
16. Burkland D, Ganapathy A, John M, et al. Near-field impedance accurately distinguishes among pericardial, intracavitary, and anterior mediastinal position. J Cardiovasc Electrophysiol. 2017;28(12):1492-1499. doi:10.1111/jce.13325
17. John M, Post A, Burkland D, et al. Confirming pericardial access by using impedance measurements from a micropuncture needle. Pacing Clin Electrophysiol. 2020;43(6):593-601. doi:10.1111/pace.13927
18. Safavi-Naeini P, Rasekh A, Saeed M, et al. D-PO05-097: Incidence of inappropriate pause detection with remote cardiac monitoring by insertable cardiac monitor. Poster presentation at HRS 2020 Science. Heart Rhythm. 2020;17(5)(suppl):S505. doi.org/10.1016/j.hrthm.2020.04.010