Advanced Techniques for Managing Complex Atrial Flutters
© 2025 HMP Global. All Rights Reserved.
Any views and opinions expressed are those of the author(s) and/or participants and do not necessarily reflect the views, policy, or position of EP Lab Digest or HMP Global, their employees, and affiliates.
EP LAB DIGEST. 2025;25(4):1,14-15,18.
Tiffany Y Hu, MD,1 and Peter A Santucci, MD2
1Assistant Professor of Medicine, Electrophysiology, Division of Cardiology, Department of Internal Medicine, Loyola University Medical Center, Maywood, Illinois; 2Professor of Medicine, Electrophysiology, Division of Cardiology, Department of Internal Medicine, Loyola University Medical Center, Maywood, Illinois
Complex atrial flutters (AFLs) have become increasingly common and can be difficult to suppress with either medications or ablation. These are predominantly encountered in patients with atrial conduction abnormalities and/or scar, either from pathology or following prior catheter ablation or cardiac surgery. These atypical (non-cavotricuspid isthmus [CTI] dependent) AFLs can be recurrent or incessant. In this article, we will discuss the pathophysiology and ablation strategies for managing complex macroreentrant AFLs.
Atypical AFLs are most frequently due to macroreentry within either the right or left atrium, but may involve both (biatrial). Post-ablation (or post-MAZE) AFLs include reentry around an identifiable central anatomic boundary, which may include intact or recovered wide area circumferential ablation (WACA) of the pulmonary veins (PVs), the mitral annulus (MA) or the posterior wall as boundaries.1 Also, ablation guided by the targeting of spatiotemporal dispersion can result in organized atrial arrhythmias.2 For right-sided AFLs, it is important to note that in the setting of prior cardiac ablation or MAZE, CTI-dependent flutter may not have the typical sawtooth appearance on 12-lead electrocardiogram.3,4 Patients who have undergone prior atriotomy may develop incisional flutter, and review of operative reports in patients who have undergone cardiac surgery, MAZE, or cardiac transplant (bicaval versus historical biatrial anastomosis) provides important clues to the potential boundaries. Lastly, AFLs may involve both atria, particularly in the setting of extensive scar and prior ablation, and may also involve epicardial connections.
Reentrant Tachycardias
Most AFLs are macroreentrant circuits around a central obstacle, typically defined as having a diameter of 2 cm or more. A combination of electroanatomical substrate and activation mapping is utilized to define the reeentrant circuit around a central conduction boundary (either anatomical, such as a valve or sleeve, or functional). Entrainment mapping may then be utilized as needed to confirm the diagnosis of AFL and to help identify the critical isthmus, distinguishing critical isthmuses from blind loops and bystander sites, as is done in other reentry cases such as ventricular tachycardia.5,6 However, entrainment results alone can be insufficient and misleading in identifying critical ablation targets. For example, in up to 18% of patients with confirmed CTI-dependent AFLs, entrainment within the CTI yielded post-pacing intervals >30 ms above the flutter cycle length.7 This may be related to slowed conduction in the setting of antiarrhythmic use (ie, amiodarone), rate-dependent slowing of conduction with faster pacing trains, and/or greater tachycardia cycle length variability. Entrainment mapping also does not specifically identify narrow or critical isthmuses. A pacing site can entrain “in” but be a part of the broad portion of an isthmus, which is a less favorable ablation target.8 It can also entrain in within a wavefront that bifurcates around a non-critical barrier and not be a critical isthmus that will terminate tachycardia. Perhaps the most dreaded response to entrainment mapping is alteration or termination of the tachycardia, which more commonly occurs with tachycardias with more variable cycle lengths and when the paced cycle length is more than 20 ms below the tachycardia cycle length.9
Single- and Dual-Loop Reentry
Historically, a distinction has been made between single- and dual-loop reentry. However, both single-loop (most CTI flutter) and dual-loop AFLs are bounded on 2 sides.10,11 This creates an overlap between single- and double-loop reentry. Frequently, the second boundary is not identified. Identifying both boundaries is useful in cases of complex AFL where the following pitfalls are commonly encountered: (1) Changing circuits during substrate/activation mapping, entrainment mapping, and ablation; (2) Failure to distinguish critical from non-critical isthmuses in the setting of significant atrial scar and multiple regions of slow conduction; and (3) Difficulty achieving isthmus block and presence of epicardial connections.
The most common single-loop reentry AFL is CTI flutter. The tricuspid annulus forms the anterior boundary, and the crista terminalis/Eustachian ridge or other caval or intercaval barrier forms the posterior boundary.10,12 While the active circuit often traverses obliquely between the anterior and posterior boundaries, ablation of the cavotricuspid isthmus effectively interrupts the circuit in all variants of CTI flutter.12
Dual-loop reentrant circuits also require 2 boundaries, making the mechanistic distinction between single- and dual-loop reentrant flutters less clear and also less critical. Commonly used nomenclature (roof, peri-mitral flutter) for dual-loop reentrant flutters identifies one loop but may not always establish the posterior or second boundary. For example, in roof flutter, the 2 boundaries are commonly the WACAs or 2 sets of PVs. In peri-mitral flutter, the anterior boundary is the MA, and the posterior boundary may involve but is not limited to the left WACA, right WACA, anterior or septal scar, or the entire posterior wall.
Two Critical Boundaries Define a Critical Isthmus
A critical boundary is a conduction barrier that is critical to maintenance of a macroreentrant circuit that can be identified by continuous sequential activation around the boundary encompassing >90% of the tachycardia cycle length.11 Non-critical boundaries, in contrast, demonstrate collision of the activation wavefront or parallel activation around both sides of the boundary. Identifying both critical boundaries allows for the identification of one or more isthmuses critical to tachycardia propagation. In this construct, ablation strategies include transecting an isthmus between the 2 critical boundaries, either with a direct line across their shared critical isthmus or by using a non-critical barrier as part of the lesion set between 2 critical boundaries.11
Often, ablation is performed across an isthmus connecting one critical boundary to the nearest conduction barrier, which is not necessarily a critical boundary, resulting in a non-critical isthmus ablation. While this may be one ablation strategy (use a non-critical boundary as a means of creating a line between 2 critical boundaries), failure to recognize this can result in confusion (either having no effect or eliciting a seeming “change” in tachycardia) and/or abandonment of the lesion set, when a simple connection of this barrier to the posterior or secondary boundary will result in successful termination and treatment of the AFL. When an isthmus between 2 critical boundaries was targeted for ablation, AFL was terminated in 96% of patients.11
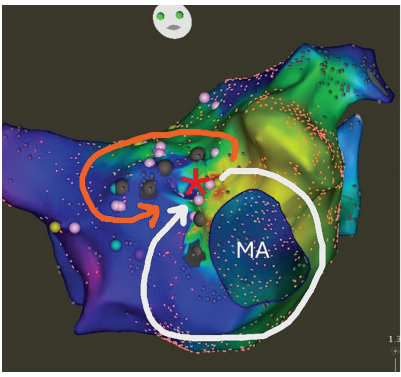
Mitral Lines
The MA commonly forms one critical boundary in atypical AFLs, and lateral or anterior mitral isthmus lines are the most common ablation line performed post PVI.13 Lateral (MA to left inferior PV) or anterior/anterolateral (MA to right or left superior PV) have different advantages and pitfalls. Posterolateral mitral lines are shorter in length; however, the coronary sinus (CS) serves as an epicardial connection as well as a heat sink, which can reduce ablation efficacy.14 In over 60% of patients, epicardial ablation within the CS may be required to achieve isthmus block.13 Vein of Marshall ethanol infusion in addition to catheter ablation increases achievement of peri-mitral block, reduces atrial fibrillation (AF) burden, and increases likelihood of freedom from AF during 12-month follow-up.15 An alternative to the posterolateral line is a superolateral mitral isthmus line targeting the thinnest segment of the mitral isthmus, from the left superior PV to the MA just below the base of the left atrial appendage (LAA). This line typically does not require CS ablation, but may carry a 5% rate of pericardial tamponade.16
Anteromedial lines may be considered, particularly when there is anterior wall scar that can be incorporated into the lesion set. In the absence of anterior scar, it can be difficult to achieve bidirectional block in these longer lines. Additionally, medial lines, even when endocardial block is achieved, may result in shunting over the right atrium (RA) with ongoing tachycardia, especially in the presence of epicardial connections.14 Utilizing both anteromedial and lateral mitral lines can risk electrical isolation of the LAA and is generally not advised.14 Anterolateral lines (left superior PV to 10 o’clock on the MA) are sometimes utilized in addition to anteromedial lines, though they risk injury to the sinoatrial artery branch of the left circumflex artery.14,18
Regardless of these anatomic considerations, consistent successful termination and management of peri-mitral flutters requires delineation of the posterior boundary in accordance with the earlier discussion and ensuring that the line connects to a posterior structure that is fully or part of the posterior critical boundary.
Figure 1 shows the map of a patient with atypical AFL. CS activation was distal to proximal, consistent with a left-sided circuit, for which transseptal access was obtained for high-density activation mapping. Clockwise activation around the MA was demonstrated. In this case, the PVs were not a part of the posterior boundary. Rather, an anterior wall boundary was identified with counterclockwise activation around this. As the anterior and posterior boundaries of the macroreentry circuit were evident from the activation mapping, entrainment mapping was not required. The narrow isthmus between the mitral valve and anterior scar was targeted with radiofrequency ablation with termination of tachycardia and demonstration of block.
In Figure 2, complex clockwise activation is seen around the MA plus septal scar in a patient with severe septal scarring. The right PVs served as the posterior boundary, which excluded the roof or lateral mitral isthmus as critical, and led to successful ablation of a small septal channel that was critical to this flutter.
Biatrial Flutters
Biatrial flutters can initially be more challenging to identify, and come in 3 variants. One variant is characterized by 2 different independent loops, such as concomitant CTI and peri-mitral flutters. In these, the faster circuit may entrain the other so that the 2 chambers appear to be in sync. In another variant, the flutter is predominantly within one chamber, but the contralateral chamber serves as an “epicardial shunt.” This may be seen, for example, with ablation of a line across the septum in a peri-mitral flutter, with slowing but not termination of tachycardia as the activation shunts into the RA and reenters the LA after working around the blocked septal line. In a third less common variant, the flutter changes back and forth between the LA and RA in a continuous but variable manner.
A biatrial flutter should be considered when mapping of both atria demonstrates 2 distinct circuits and when widely disparate sites (eg, both the RA and distal CS) entrain in. It should also be suspected when ablation of an atypical flutter in one chamber results in a “change” in flutter to the opposite chamber, as well as when there are spontaneously changing flutters.
An effective ablation strategy depends on the particular type of biatrial flutter. In many cases, ablation of the 2 independent loops is most effective. When there is shunting across both atria in a single large loop, identifying the 2 complex boundaries will allow effective ablation of a critical isthmus, typically within one atrium, avoiding a septal line.
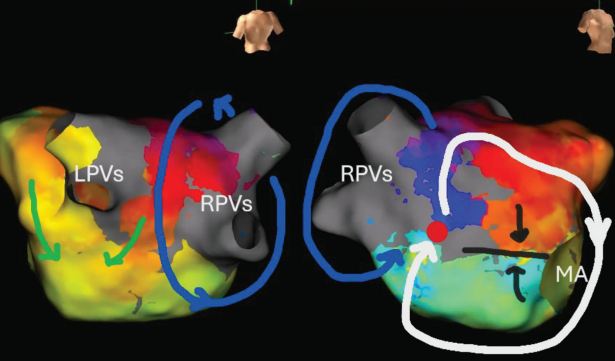
Pulsed Field Ablation
The safety and efficiency of pulsed field ablation (PFA) on the posterior wall may result in a greater incidence of posterior wall isolation attempts during first-time AF ablation. Due to the acute effects of PFA on electrograms, post-ablation mapping is not a reliable indicator for irreversible electroporation and ablation success. Therefore, one can imagine the potential reentrant circuits a partially ablated posterior wall or larger than anticipated PVI may predispose to.19 Further studies are needed to clarify the long-term significance of these lesion sets in complex flutters.
Disclosure: The authors have completed and returned the ICMJE Form for Disclosure of Potential Conflicts of Interest. Dr Santucci reports speaker fees and travel support for conference (speaker) from Abbott; Dr Hu reports a travel scholarship from the Heart Rhythm Society.
References
1. Lim TW, Koay CH, See VA, et al. Single-ring posterior left atrial (box) isolation results in a different mode of recurrence compared with wide antral pulmonary vein isolation on long-term follow-up: longer atrial fibrillation–free survival time but similar survival time free of any atrial arrhythmia. Circ Arrhythm Electrophysiol. 2012;5(5):968-977. doi:10.1161/CIRCEP.111.970293
2. Bahlke F, Englert F, Popa M, et al. First clinical data on artificial intelligence-guided catheter ablation in long-standing persistent atrial fibrillation. J Cardiovasc Electrophysiol. 2024;35(3):406-414. doi:10.1111/jce.16184
3. Chugh A, Latchamsetty R, Oral H, et al. Characteristics of cavotricuspid isthmus–dependent atrial flutter after left atrial ablation of atrial fibrillation. Circulation. 2006;113(5):609-615. doi:10.1161/CIRCULATIONAHA.105.580936
4. Akar JG, Al-Chekakie MO, Hai A, et al. Surface electrocardiographic patterns and electrophysiologic characteristics of atrial flutter following modified radiofrequency MAZE procedures. J Cardiovasc Electrophysiol. 2007;18(4):349-355. doi:10.1111/j.1540-8167.2007.00761.x
5. Barbhaiya CR, Kumar S, Ng J, et al. Overdrive pacing from downstream sites on multielectrode catheters to rapidly detect fusion and to diagnose macroreentrant atrial arrhythmias. Circulation. 2014;129(24):2503-2510. doi:10.1161/CIRCULATIONAHA.113.008494
6. Kumar S, Tedrow UB, Stevenson WG. Entrainment mapping. Card Electrophysiol Clin. 2017;9(1):55-69. doi:10.1016/j.ccep.2016.10.004
7. Vollmann D, Stevenson WG, Luthje L, et al. Misleading long post-pacing interval after entrainment of typical atrial flutter from the cavotricuspid isthmus. J Am Coll Cardiol. 2012;59(9):819-824. doi:10.1016/j.jacc.2011.11.023
8. Delacretaz E, Ganz LI, Soejima K, et al. Multi atrial macro-re-entry circuits in adults with repaired congenital heart disease: entrainment mapping combined with three-dimensional electroanatomic mapping. J Am Coll Cardiol. 2001;37(6):1665-1676. doi:10.1016/s0735-1097(01)01192-5
9. Barbhaiya CR, Kumar S, Ng J, et al. Avoiding tachycardia alteration or termination during attempted entrainment mapping of atrial tachycardia related to atrial fibrillation ablation. Heart Rhythm. 2015;12(1):32-35. doi:10.1016/j.hrthm.2014.09.002
10. Kalman JM, Olgin JE, Saxon LA, Fisher WG, Lee RJ, Lesh MD. Activation and entrainment mapping defines the tricuspid annulus as the anterior barrier in typical atrial flutter. Circulation. 1996;94(3):398-406. doi:10.1161/01.cir.94.3.398
11. Santucci PA, Bhirud A, Vasaiwala SC, Wilber DJ, Green A. Identification of 2 distinct boundaries distinguishes critical from noncritical isthmuses in ablating atypical atrial flutter. JACC Clin Electrophysiol. 2024;10(2):251-261. doi:10.1016/j.jacep.2023.09.024
12. Santucci PA, Varma N, Cytron J, et al. Electroanatomic mapping of postpacing intervals clarifies the complete active circuit and variants in atrial flutter. Heart Rhythm. 2009;6(11):1586-1595. doi:10.1016/j.hrthm.2009.08.010
13. Chae S, Oral H, Good E, et al. Atrial tachycardia after circumferential pulmonary vein ablation of atrial fibrillation: mechanistic insights, results of catheter ablation, and risk factors for recurrence. J Am Coll Cardiol. 2007;50(18):1781-1787. doi:10.1016/j.jacc.2007.07.044
14. Hamoud NS, Abrich VA, Shen WK, Mulpuru SK, Srivathsan K. Achieving durable mitral isthmus block: challenges, pitfalls, and methods of assessment. J Cardiovasc Electrophysiol. 2019;30(9):1679-1687. doi:10.1111/jce.14079
15. Valderrábano M, Peterson LE, Swarup V, et al. Effect of catheter ablation with vein of Marshall ethanol infusion vs catheter ablation alone on persistent atrial fibrillation: the VENUS randomized clinical trial. JAMA. 2020;324(16):1620-1628. doi:10.1001/jama.2020.16195
16. Maurer T, Metzner A, Ho SY, et al. Catheter ablation of the superolateral mitral isthmus line: a novel approach to reduce the need for epicardial ablation. Circ Arrhythm Electrophysiol. 2017;10(10):e005191. doi:10.1161/CIRCEP.117.005191
17. Lai Y, Guo Q, Sang C, et al. Revisiting the characteristics and ablation strategy of biatrial tachycardias: a case series and systematic review. Europace. 2023;25(3):905-913. doi:10.1093/europace/euac231
18. Cho Y, Lee W, Park EA, et al. The anatomical characteristics of three different endocardial lines in the left atrium: evaluation by computed tomography prior to mitral isthmus block attempt. Europace. 2012;14(8):1104-1111. doi:10.1093/europace/eus051
19. Magni FT, Scherr D, Manninger M, et al. Electrophysiological findings during re-do procedures after single-shot pulmonary vein isolation for atrial fibrillation with pulsed field ablation. J Interv Card Electrophysiol. 2023;66(7):1729-1737. doi:10.1007/s10840-023-01559-z